Unlike other polymeric materials used in T&D applications, silicones (also known as polydimethylsiloxanes) are not made up of a carbon to carbon backbone. Rather, they consist of repeating silicon-oxygen (siloxane) groups with two methyl groups each bonded to the silicon. As such, they differ widely from hydrocarbon-based materials.
This past contribution to INMR discussed important aspects of this dominant family of materials when it comes to electrical insulation applications.
One accepted standard abbreviation for silicones is VMQ, where Q stands for rubber having silicon and oxygen in the polymer chain while M indicates that the material mainly contains methyl groups (CH3). V stands for the vinyl groups (R-CH=CH2) that represent the material’s curable species. It is this chemical structure that makes silicones able to satsify important properties required across diverse power industry applications.
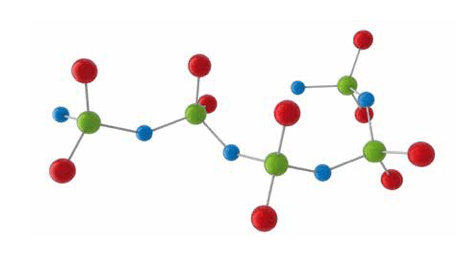
Stability Under UV Radiation
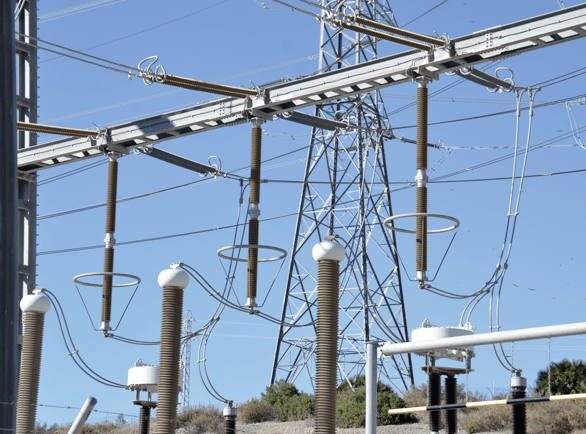
Silicones have a structure similar to organically modified quartz. The high bonding energy of the silicon to oxygen (Si-O) backbone provides stability against breakdown by UV irradiation. For example, the bonding energy of Si-O bonds is 444 kJ/mol versus only 348 kJ/mol for C-C bonds. Shortwave sunlight at 300 nm has an energy content of about 6.2×10-22 kJ (i.e. 398 kJ/mol) and can therefore cleave C-C bonds but not the Si-O bond that remains stable. Even high UV and harsh weather conditions such as found in coastal regions or deserts have little impact on this resistance property. Silicones show only slight changes in mechanical and wetting behavior, even after extended accelerated weathering testing in climatic chambers.
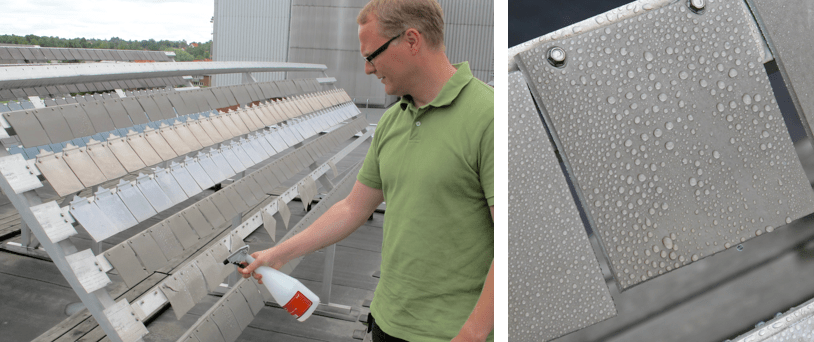
Hydrophobicity
Silicone polymers and molded components made with silicone elastomers exhibit low surface energy that does not allow water to spread out over their surface, i.e. a hydrophobic or water repellency property. This highly desirable characteristic can be evaluated by measuring wetting angle of water droplets on the surface of silicone specimens.
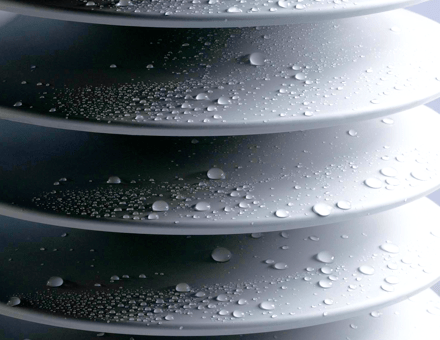
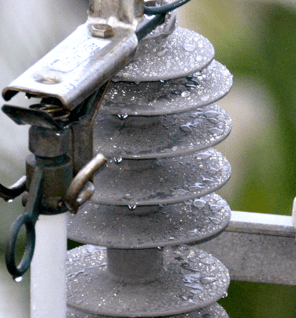
Service experience confirms that leakage current along an electrically stressed hydrophobic surface is limited to low values. Wetting performance of insulation therefore increases compared to the same surface covered with a layer of film, as long as the droplet layer of water remains mostly intact. This same characteristic also extends to polluted surfaces as a result of the hydrophobicity transfer effect. Silicone elastomer formulations contain a tiny but not negligible amount of short-chain, low molecular weight (LMW) siloxane chains. When driven by a difference in concentration between the base silicone elastomer and the pollution layer, these mobile species migrate into and encapsulate the pollution layer. In fact, the rough surface of the now hydrophobic pollution layer often shows an even higher wetting angle than the shiny surface of the clean silicone. This effect promotes sustained hydrophobicity.
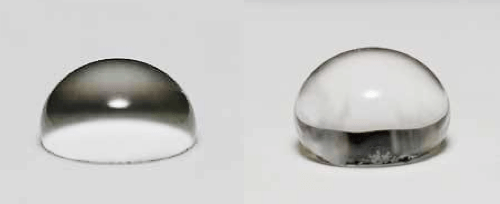
Thermal Stability
Due to chemical structure, silicones demonstrate stability even at elevated temperatures. Most silicone elastomers remain stable and elastic up to 200°C while modified products can withstand as high as 300°C. This is confirmed by tests of weight loss of different silicone elastomers (VMQ A, B and C) stored at 200°C. It is important to note, however, that thermal stability is not itself a physical property but rather requires definition of permitted changes in key performance properties, such as elongation at break, hardness, weight loss, etc., within the time period considered. For example, it has been shown that the tensile strength of a silicone elastomer exposed to 200°C in air retained 80% of its initial value even after 700h constant exposure. Standard silicone elastomers (VMQ) maintain elasticity at temperatures down to -45°C, which makes them suitable for use in cold temperatures as well. In fact, special modified silicone gels remain flexible up to -100°C.
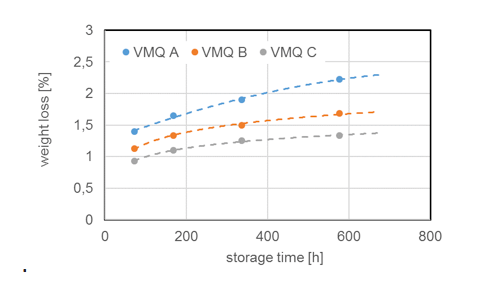
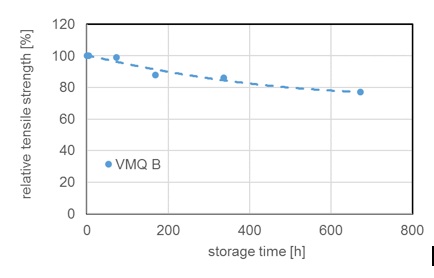
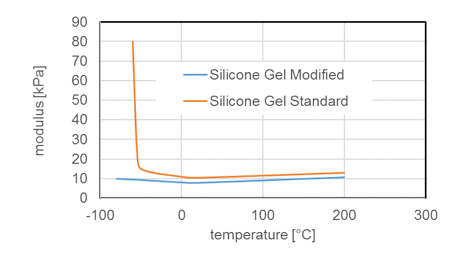
Flame Retardancy
Silicones ignite only at very high temperature and thus exhibit good flame-retardancy. Typically, silicone elastomers show either class V0 or HB under the relevant test criteria, making them particularly safe in operation. Moreover, if they do ignite, they burn without producing toxic gases so they are well suited for applications subject to strict fire safety requirements, e.g. subways, tunnels, high-rise buildings, etc.
Key Properties for Electrical Applications
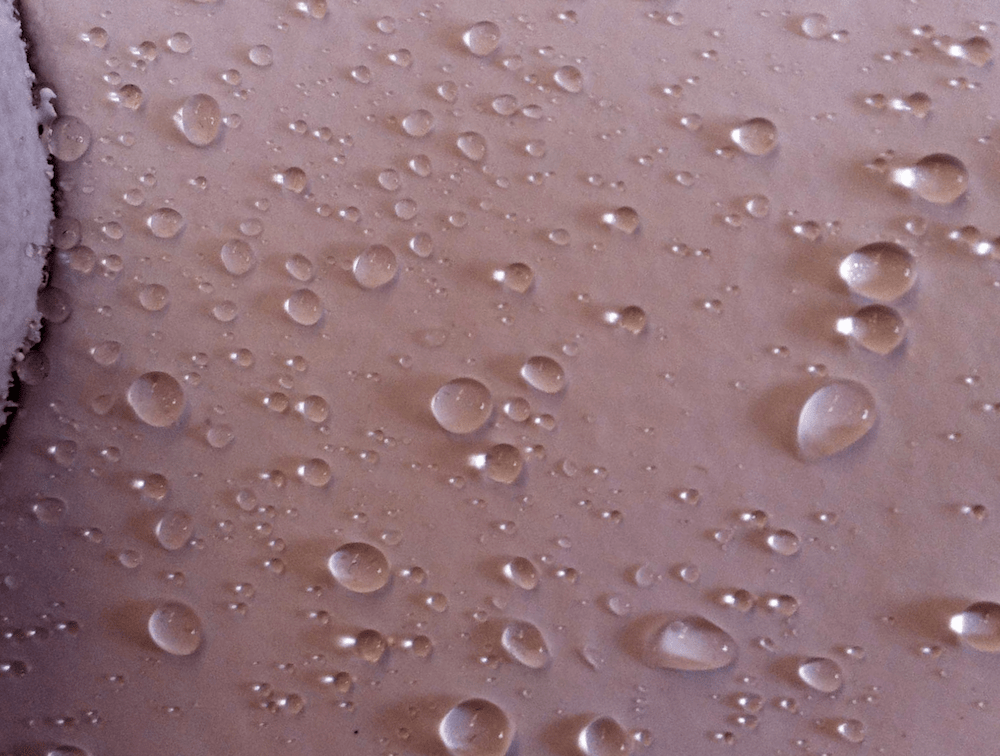
Silicones offer attractive properties for electrical applications in general but each specific application may require a somewhat different key property. For example, the original focus for insulator coatings was on water-repellancy of the RTV silicone material. Later, when composite insulators were introduced, the high UV resistance of silicone elastomers offered another advantage. Such key performance properties for various applications have remained largely the same over the years, with the possible exception of transformer fluids where the focus has shifted from low flammability to temperature resistance.
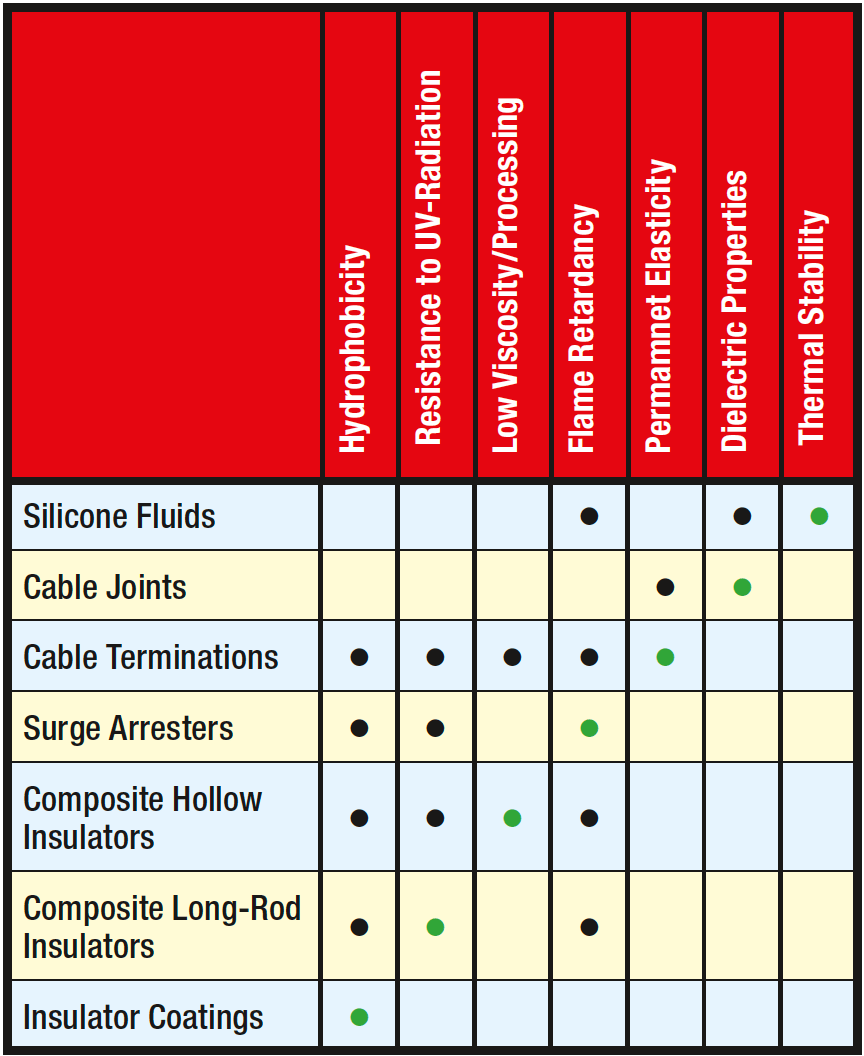
Product Groups
Silicone Elastomers
Silicone elastomers are the most important group of silicones serving the world’s transmission and distribution industry. Owing to the wide range of potential applications, they differ with respect to viscosity and curing systems and are continually undergoing further refinement to optimize production and performance. There are three major groups of these elastomers.
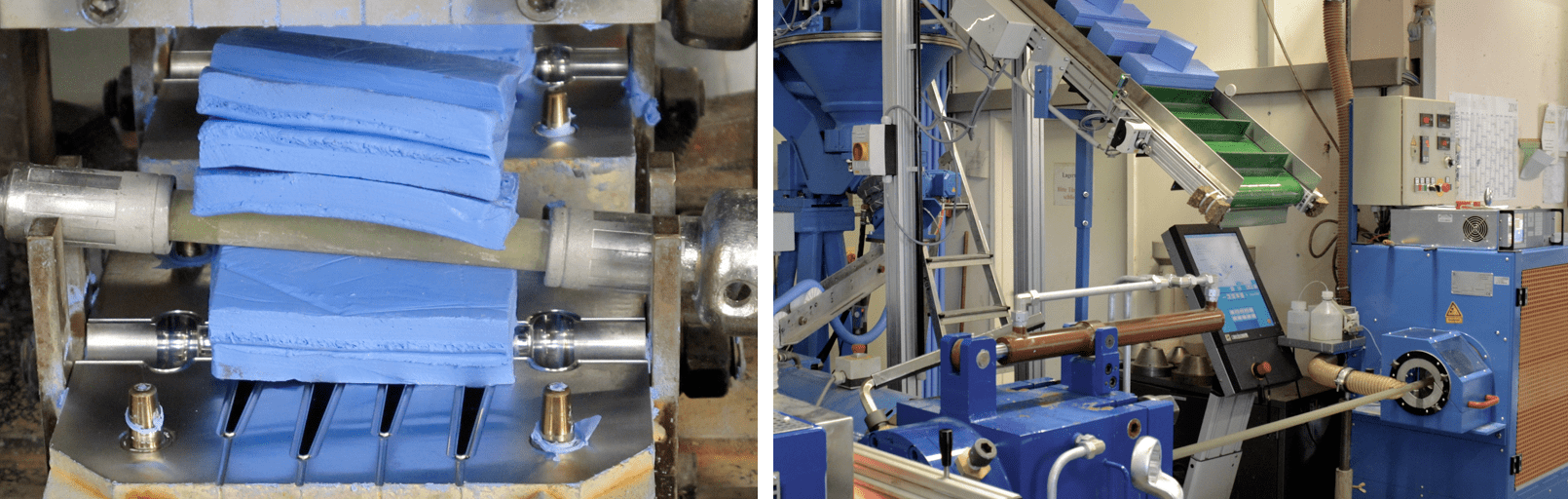
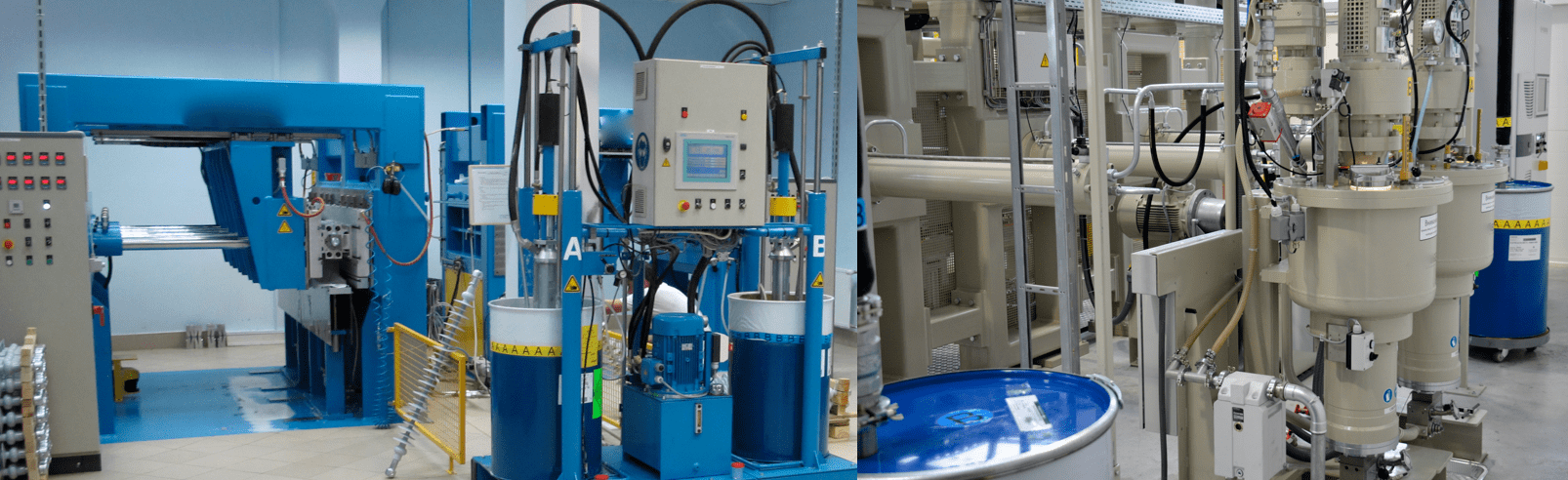
High Consistency Silicone Rubber (HCR)
These materials, with high viscosity and typically supplied as logs are used for injection molding, press molding or extrusion. HCRs are typically peroxide curing materials but one and two-component addition curing systems are also available.
Liquid Silicone Rubber (LSR)
These 2-component materials have viscosity of some 10,000 to 1,000,000 mPa and are supplied in drums or similar. They are either injection molded or directly filled into a mold using meter-mixing devices (low pressure mold filling or LPMF). All LSRs are addition-curing materials.
RTV Silicones
Silicones that often have low viscosity and whose formula allows cross-linking at comparatively low temperature are grouped under Room Temperature Curing materials (RTV). While the ‘V’ stands for vulcanization, crosslinking of these materials occurs either by polyaddition or polycondensation. RTV materials can be 2-component (rubbers) or 1-component (e.g. coatings).
Silicone Gels
Silicone gels are insulating materials that have the potential to replace liquids or elastomers, depending on application. They pose less risk of leakage than fluids yet perfectly fill hollow spaces, even of complex shape. These gels, typically used as insulating compounds for cable accessories, etc., are pourable, addition curing, two-component silicones that cure to soft, gel-like products. The unmixed components usually demonstrate low viscosity with excellent pouring properties. The cured silicone gel generally adheres well to all substrates in contact with it.
Silicone Fluids & Pastes
Silicone fluids have proven themselves as coolants and insulating materials in modern transformers due to outstanding temperature resistance. A further important application is in the area of high voltage cable accessories. Silicone fluids are non-reactive, linear PDMS with chain length from 2 to well over 1000 Si atoms. Adding thickeners makes it possible to produce silicone pastes, used for example as lubricants in the electrical industry.
Curable Silicone Compounds
The primary components in curable silicone compounds are polysiloxanes. These are produced by converting quartz (SiO2) into silicon metal (Si) using a metallurgical process, followed by transformation into chlorosilanes based on the Müller-Rochow process. Silanes are then converted into polysiloxanes by hydrolysis technology. The properties of polysiloxanes and the products made from them depend greatly on structure of their chemical backbone and attached functional groups. Apart from these polysiloxanes, silicone compounds often contain reinforcing fillers, cross-linkers and additives to achieve desired processing and product performance. Conversion of the uncured compound into an elastic state – known as curing or cross-linking – involves formation of covalent bonds between polymer chains. Depending on class of silicone rubber, peroxides, silanes or SiH-containing siloxanes are required for such cross-linking to occur.
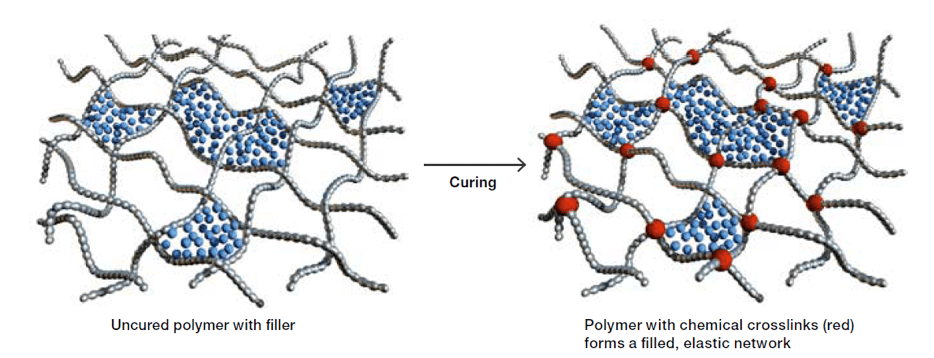
Fillers reinforce the elastic silicone network and help adjust rheological properties. The nature, composition and quantity of these fillers are critical in determining the properties of the uncured and cured rubber. For example, the most effective reinforcing filler is pyrogenic silica (fumed silica) with high BET surface. Additional fillers such as quartz, alumina trihydrate (ATH) and carbon black might also be used to achieve the desired thermal stability, media resistance or electrical properties.
The suitability of a compound for a particular processing method is dictated by its rheological properties, i.e. viscosity, which describes its flow characteristics. Higher viscosity means less pourable. Viscosity of Newtonian fluids such as polysiloxanes is independent from shearing. By contrast, compounds made from polysiloxanes and fillers show non-Newtonian behavior, whereby there is significant decrease in viscosity with increased shearing. Rheological behavior of uncured silicone rubber is important since it facilitates manufacture of electrical components by extrusion or injection molding. The significant shear-thinning effect as well as solubility of air must also be taken into account when processing silicone rubber. Settings such as temperature, pressure and flow control in state-of-the-art molding equipment enable reliable production of large volume pieces e.g. cable accessories as well as articles having complex geometry and high precision.
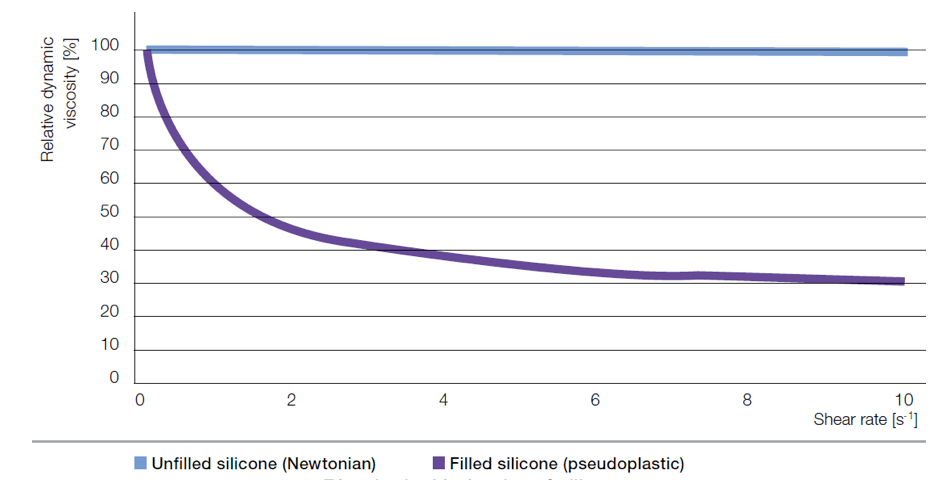
Various technologies exist to cross-link polymers such as silicone elastomers with three having achieved broad commercial application:
1. Condensation-Curing Silicones
Condensation-curing silicone rubbers for electrical applications come as ready-to-use one-component compounds. Cross-linking technology here is based on reaction between Si-OH groups and/or hydrolysable Si-X groups in the presence of moisture. Right after application, the material starts to cross-link when in contact with the moisture in air. Depending on nature of the cross-linker, small amounts of acetic acid, amine or neutral by-products such as alcohol are released during curing.
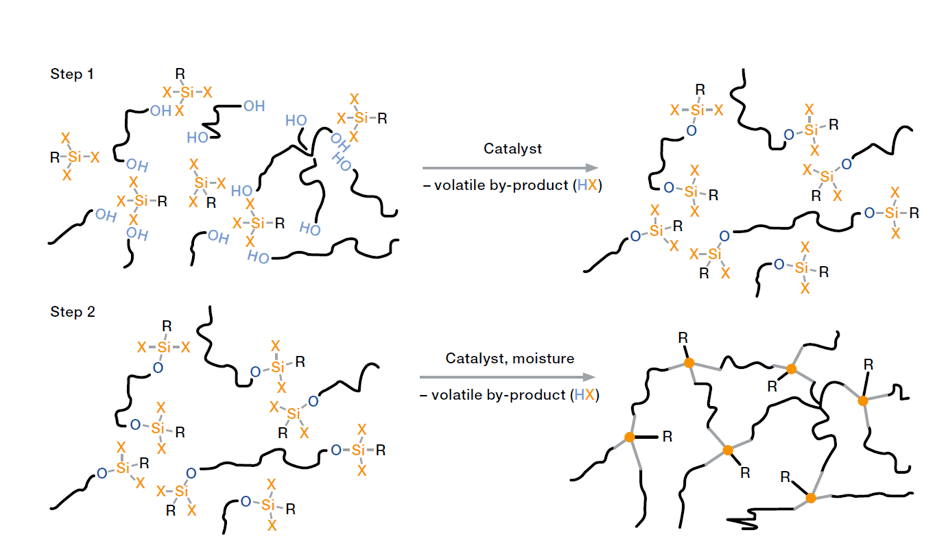
Cross-linking starts with formation of a skin on the rubber surface and gradually progresses into the compound. Curing rate of these silicones is limited by the speed of diffusion of moisture into the compound. Therefore, monitoring and possibly controlling temperature and relative humidity is recommended to ensure reliable curing. As ready-to-use systems that are not sensitive to inhibition by other substances, condensation-curing silicone rubbers show easy handling and processing properties. Because of good adhesion to a variety of substrates, e.g. metal parts, ceramics, plastics and cured silicone rubber parts, such silicones are ideal for sealing, bonding and coating. High diffusion rate of moisture through thin layers of silicone rubber as well as ease of handling and excellent adhesion properties have led to development of silicone coatings e.g. for glass and ceramic insulators as well as other components. For short cycle times, however, production of large volume parts and also for special properties (e.g. heat and media resistance), applicability of condensation-curing RTV-1 technology is limited and other cross-linking systems are preferred.
2. Peroxide Induced Cross-Linking
Peroxide curing involves use of organic peroxides. At elevated temperatures, these decompose to form highly reactive radicals that chemically cross-link the polymer chains leading to highly elastic, three-dimensional networks. For commercially available compounds, peroxide-induced curing is used for so-called high consistency rubber. They typically contain polymers with a high molecular weight and relatively long polymer chains.
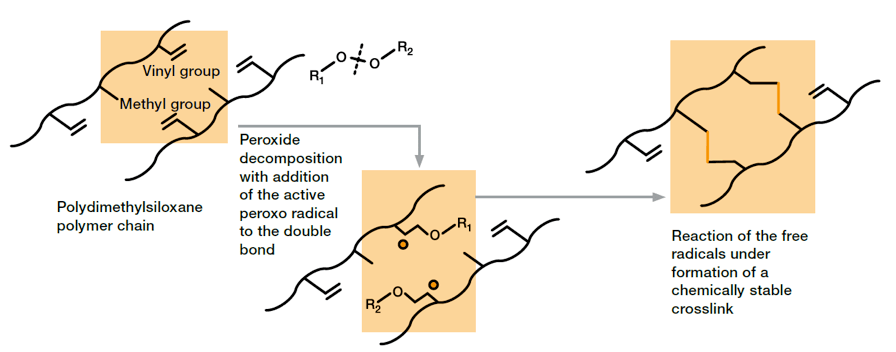
Several peroxides have proven suitable for crosslinking of silicone rubber. Beside crosslinking-temperature range, which is characteristic for each peroxide and typically ranges from (100 – 200)°C, the radicals formed during vulcanization differ regarding their tolerance to atmospheric oxygen. However, suppliers of silicone rubber have several peroxide systems in their portfolio, which allows them to provide material solutions for customarily applied processing methods such as extrusion, press molding and injection molding. Some applications require additional post-curing of the peroxide-cured silicone rubber in order to improve mechanical properties (e.g. low compression set) or to remove volatile cleavage products. Excellent mechanical strength of the products, good resistance regarding inhibitors or contaminations (compared to platinum-curing) in combination with proven and technically mature processes, which have been in use for decades, led to a wide range of application in the market.
Platinum-Catalyzed Hydrosilylation (Addition Curing)
Platinum-catalyzed addition curing, known as hydrosilylation, is a reaction between Si-H rich crosslinkers and vinyl-functionalized polysiloxanes. Addition curing is applicable to both solid and liquid silicone rubber. The reaction starts even at low temperature as soon as the catalyst is mixed with the other components. However, by adding inhibitors the pot life can be adjusted to some hours, days and for one-component ready-to-use systems even months. Addition curing enables crosslinking at room temperature as well as temperature-induced heat curing systems.
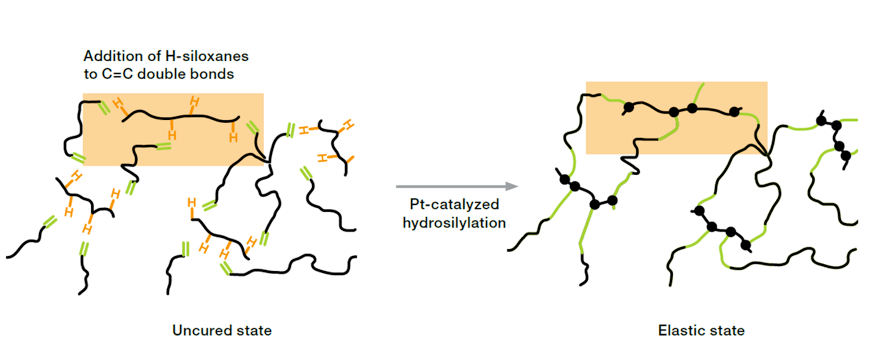
The outstanding activity of platinum enables catalyst loadings in the ppm-range. For fabricators applying addition curing products it is important to know that even traces of platinum might trigger crosslinking reactions (platinum contamination). Because of the low platinum content even small amounts of catalyst poisons can inhibit the catalyst, especially amine- and sulfur-containing compounds. Separation of production lines, e.g. from organic rubber, and proper cleaning of manufacturing equipment is highly recommended in order to prevent cross-contamination. Despite above-mentioned behavior of Pt-curing compounds, the application of addition curing is state-of-the-art, since this technology creates several advantages regarding manufacturing and properties of the product:
Raising temperature significantly increases the rate of crosslinking relative to peroxide curing systems. Well-adjusted balance and concentration of Pt-catalyst and inhibitors together with proper settings of the manufacturing equipment allow shorter cycle times and energy efficient production even of large volume parts, which is just one example for the special challenges in T&D-industry. Good demolding properties and dry surface of cured rubber parts further improve manufacturing processes.
Addition curing generally facilitates formation of homogeneous, well-defined silicone rubber networks with outstanding mechanical performance (e.g. tear strength, elasticity), whereas selectively loose crosslinking of polysiloxane-chains enables the formation of non-bleeding silicone gels with exceptional sealing properties.
Unlike peroxide curing, platinum-catalyzed crosslinking does not liberate volatile by-products.
Challenging and versatile demands in T&D require a large portfolio of materials and technologies in the field of silicone elastomers such as non-bleeding gels for sealing applications, well adhering coatings, liquid and solid silicone rubber for large volume parts or flame retardant rubber for safety cables, just to mention some of the systems applied. Polymer structure and functionality, amount and nature of the filler as well as crosslinking density and applied curing method gives silicone elastomers an outstanding variety of properties. A deep understanding of the interactions between all ingredients is essential to achieve good storage stability, reliable processability and a product performance, which is well adjusted to the desired application.