The 70 kV Parakan-Kadipaten overhead transmission line in Indonesia is among those at the country’s TSO – PLN UIT JBT – that has experienced a significant frequency of disturbances. While programs have recently been instituted to enhance its protection system, these proved inadequate in mitigating the severity of lightning disruptions. A Proof of Concept (POC) was therefore conducted in 2022 to evaluate the efficacy of applying externally gapped lightning arresters (EGLAs) to resolve this problem, based on findings discussed in CIGRE TB 855.
This edited contribution to INMR by Rizally Priatmadja and other engineers at PT PLN (Persero) in Indonesia provides an overview of the preparatory and design phases, followed by an analysis of the installation process and an evaluation of performance spanning several months of operation.
Background
PLN UIT JBT manages a transmission network of OVER 13,000 kmc and over 19,000 towers divided into 619 circuits covering Central and West Java. Most disturbances are dominated by lightning. Figs. 1 and 2, for example, show the transmission line’s outage record from 2017 to 2022, which accounts for an average of 43% of total accumulated disturbances and an average of 90% of total natural disturbance. Based on the Forced Outage Information System (FOIS) application record, 90% of lightning disturbances occur on 70 kV transmission lines. And one of the overhead lines with the highest total accumulated outages is the 70 kV Parakan-Kadipaten OHL.
This line is located in a region with isokeraunic levels of from 25 to 50 thunderstorm days per year. Table 1 from the Forced Outage Information System (FOIS) at PLN provides data on its fault records, which saw 20 fault occurrences caused by lightning between 2016 and June 2023. During this period of 7.5 years, seven towers were impacted by lightning strikes, making this the most prevalent incident. Various measures were undertaken to decrease frequency of outages, including implementing a multirod grounding (MRG) system and reducing tower inductance. Nevertheless, there was still a notable rise in fault incidents over the following several years.
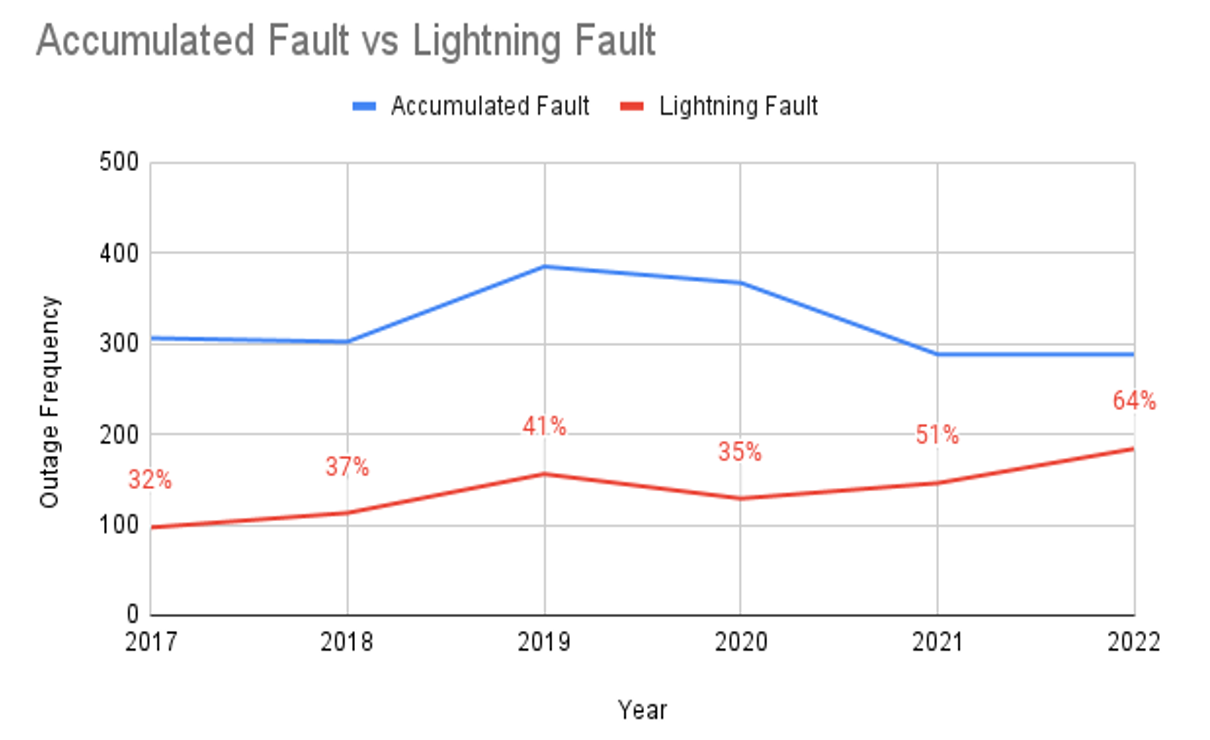
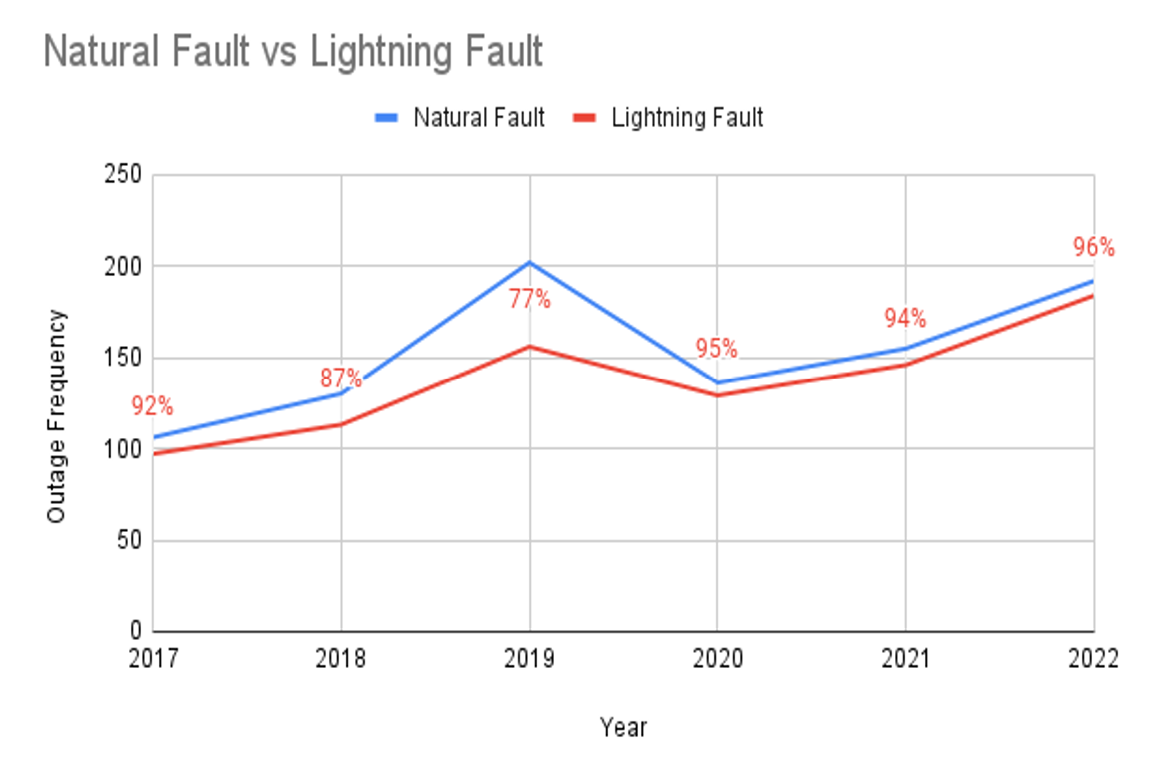
Responding to these issues, at the end of 2022 PT PLN (Persero) started work in partnership with local arrester supplier, PT Rahmat Kurnia Abadi, to evaluate and improve the lightning performance of transmission lines. Starting Dec. 9, 2022, about 79 externally gapped line arresters (EGLAs) were installed along the transmission line with poorest lightning performance and in specific configurations. From that date on, only 3 outages due to lightning were recorded on this line, proving the effectiveness of this protection system.
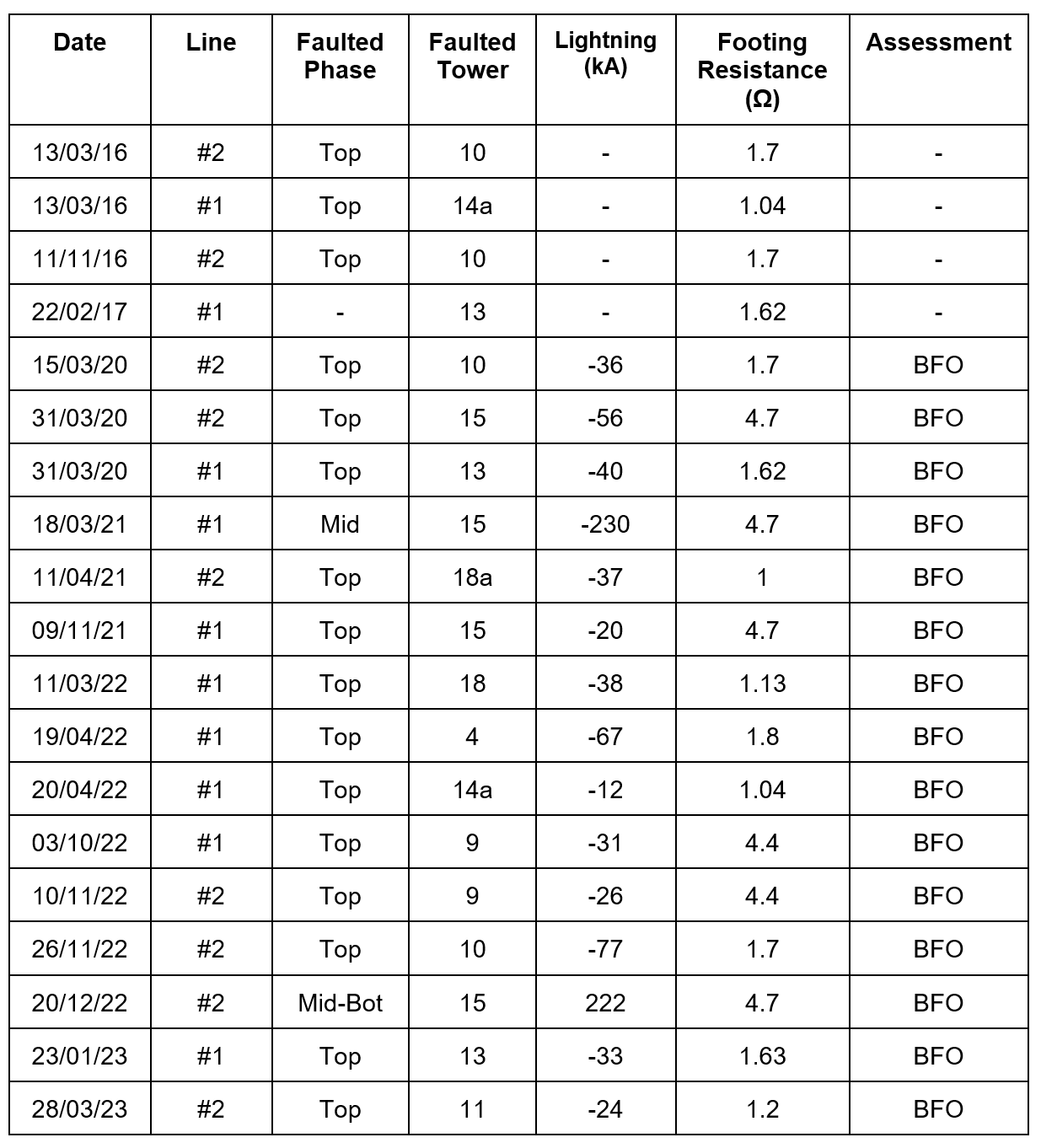
The discussion below reviews the transmission line lightning performance studies and evaluation carried out in this partnership project. Methods are presented to select arrester characteristics and define the quantity and the optimized location along the line. Field experience obtained during the first 6 months and the line performance/reliability of the system after EGLA application are compared with performance before arrester installation.
Literature Review
a. Shielding Failure Analysis
In order to investigate preventative measures such as shield wire, it is important to analyze the parameters of striking distance and exposure radius. To ensure protection of an overhead power line, it is imperative that the exposure radius does not surpass the striking distance. In the conventional electromagnetic model of conductors and shield wires, the variable, rg denotes radius of the lightning strike from the point of contact with earth while Dc represents the distance at which shielding failure occurs. On the other hand, rc represents the radius of a lightning strike of a specific magnitude on the phase conductor and shield wire. Based on the references provided, a number of striking distance equations have been identified.
The present study utilized the Brown-Whitehead constants as outlined below:
Moreover, Ng is the ground flash density, whereas the yearly flash incidence, NL, is a measure of flash occurrences on the shield wire per 100 km.
The variable b represents distance between shield wires or the outermost phase conductors, whereas the variable h represents height of the tower. In order to approximate the annual occurrences of flash incidents on each phase conductor, shielding failure rate (SFR) is utilized.
In this context, the variable “l” represents the length of the line, “IMSF” is the maximum lightning current that can occur before shielding failure, and “f(I)” represents the probability of peak lightning current occurrence. Moreover, considering that Ic represents the crucial stroke current in kiloamperes (kA), the total rate of shielding failure flashover (SFFOR) to the phase conductor can be determined.
When a flashover is triggered by a lightning strike, the voltage at the highest point of the transmission tower will reach its maximum value at the peak of the lightning current. Therefore, the voltage at the top of the tower (VTT) can be expressed in terms of the lightning current, I(t).
(dI(t))/dt is a measure of lightning steepness, LT represents inductance of the tower and RE signifies tower footing resistance. To compute LT, it is necessary to obtain the values of the tower surge impedance, ZT, tower height, HT, and the speed of light, c.
The tower, functioning as a variable-impedance transmission line, partitions the tower section into a series of parts with distinct surge impedance values that correspond to the height and average radius. Hence, overall surge impedance, ZT, of the tower can be determined by summing the surge impedance of each individual part of the tower.
b. Back-Flashover Analysis
Implementation of effective shield wire protection measures has the potential to result in an inadvertent discharge towards the phase conductor. The occurrence of a lightning strike on the shield wire or tower structure results in substantial potential differences across the line insulator. Consequently, when the potential surpasses the insulation strength, backflashover occurs. The anticipated annual backflashover rate (BFOR) per 100 kilometres is calculated as follows:
The probability, denoted as Ic, of the initial stroke current surpassing the critical current, Ic, can be approximated as:
Profile SUTT 70 kV Parakan-Kadipaten
The 70 kV Parakan-Kadipaten OHL has operated for 50 years, delivering energy from the Parakan Hydropower Plant with a total capacity of 10 MW. In its initial period of operation, this conductor was operated at 30 kV. Then, in 1973, the line was operated at 70 kV. Average distributed load is 113.25 amperes with a maximum capacity of 380 amperes. To monitor the line’s lightning performance against potential lightning disturbances, PLN has four LDS sensors installed in locations across the island of Java.
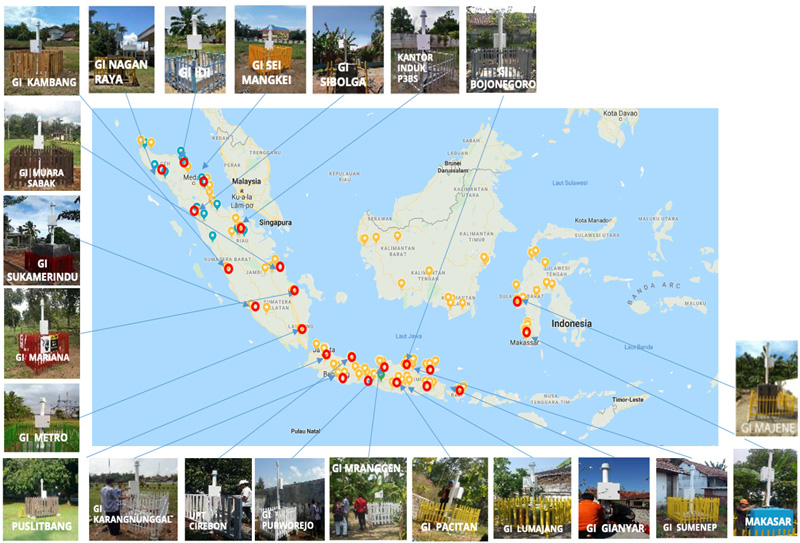
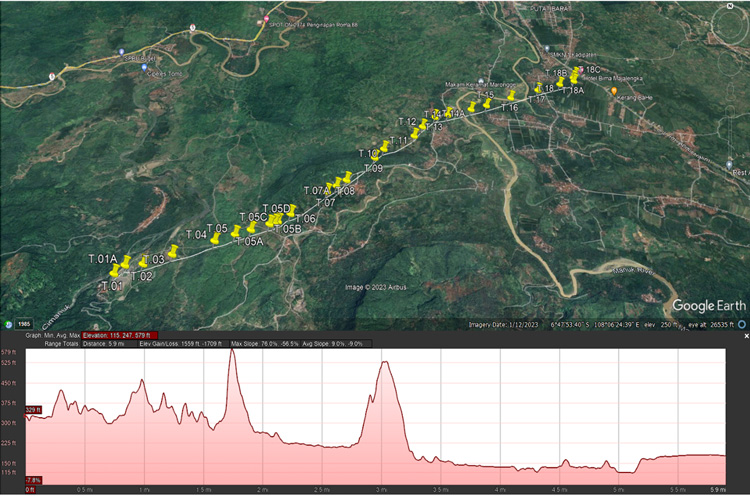
One of the reasons for choosing this transmission line for this project was the ease and flexibility of line shutdown during the installation process. Since a special placement study process does not accompany initial installation, it is possible that in the future, if there is a placement optimization process, this can be carried out flexibly.
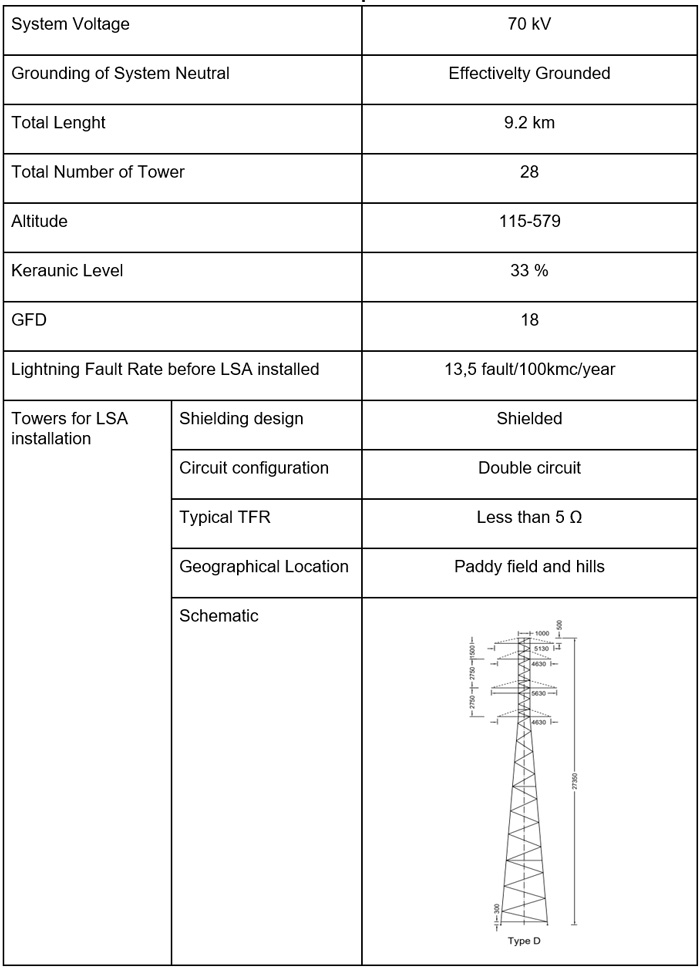
Lightning Characteristics & Statistics
Lightning characteristics were studied across the sub-transmission line over the period 2017 to 2022, including peak current statistics, flash density and periodic flash distribution. Over the last 6 years, 2691 lightning occurrences occurred along the 70 kV Parakan-Kadipaten OHL, with 92.87% being negative in polarity. Fig. 5 depicts distribution of peak current frequency from 2017 to 2022 on both the negative and positive polarity lightning flashes. As shown, most currents over the past 6 years occurred between 10 and 22 kA.
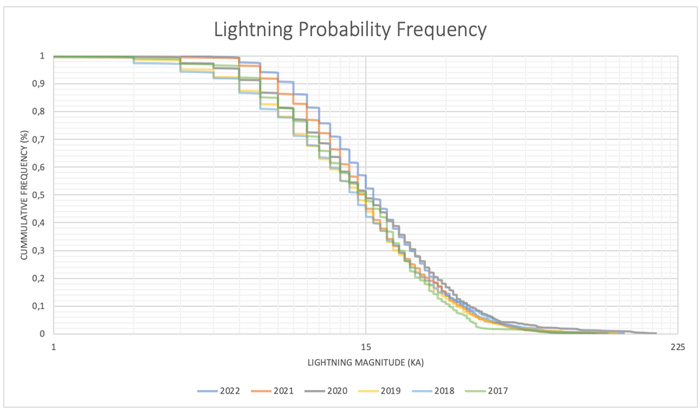
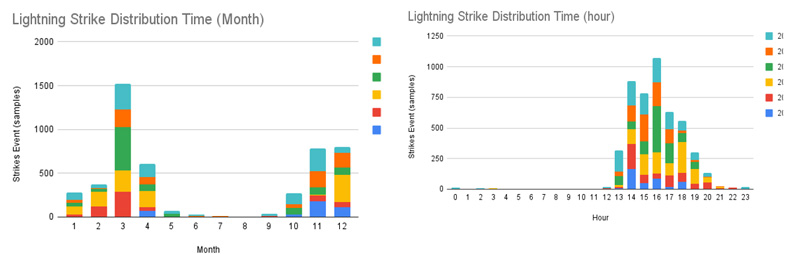
(a) monthly distribution (b) hourly distribution.
In general, total accumulation based on 2017-2022 strike data, showed that lightning strikes on this line reached their peak in March. Strikes occurred in the range of 01 pm and 12 pm and the highest number occurred between 2 pm and 4 pm.
Lightning Performance Review
Two approaches were tried to assess impact of frequent disturbances on the Parakan-Kadipaten 70 kV SUTT area. The first was value of tower leg grounding in that section. It is known that grounding resistance of the tower legs plays a significant role in providing lightning protection when a back-flashover occurs. Fig. 7 provides results of tower foot grounding measurements in the Parakan-Kadipaten 70 kV SUTT area.
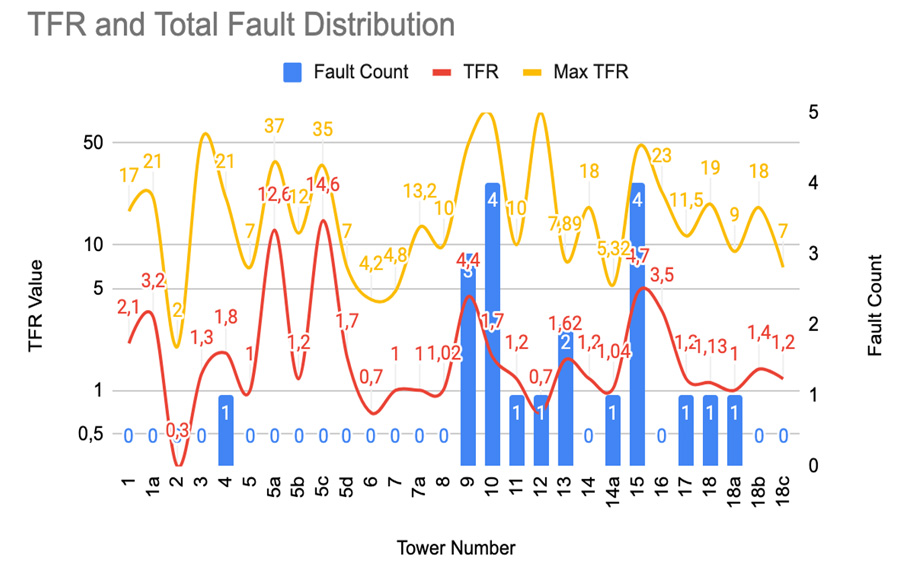
In addition, current methodology relies on historical strike data from PLN’s Lightning Detection System (LDS) program. Lightning strike data utilized in this study covered the period from 2017 to 2022. The next step involved acquiring disturbance history data and disseminating findings of post-disturbance inquiries to illustrate the areas most impacted. Utilizing such data, it became possible to analyze the features of lightning disturbances, specifically for the 70 kV Parakan-Kadipaten OHL segment.
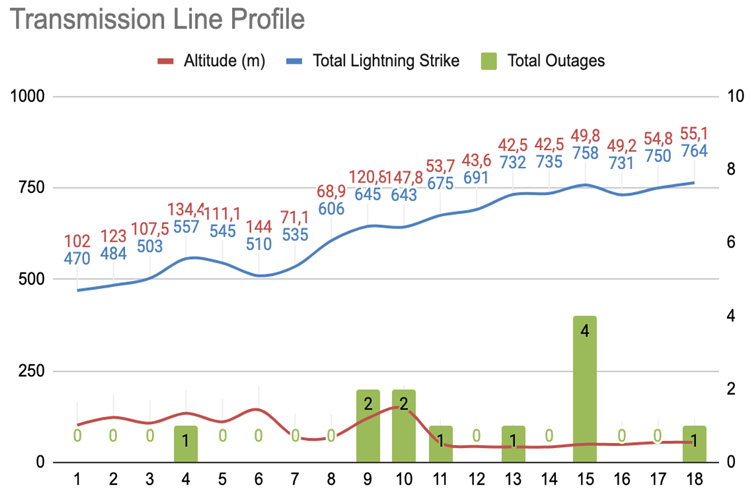
The relationship between altitude of transmission line towers and occurrence of disturbances is not directly influenced by geographic conditions or intensity of lightning strikes on the transmission line section. Disruptions experienced during period from 2018 to 2023 exhibited a direct correlation with the elevated frequency of lightning strikes observed on each individual tower, i.e. the higher the frequency of lightning strikes, the greater the likelihood of disturbances occurring. For example, rice fields are typically characterized by a greater incidence of lightning strikes and a higher prevalence of lightning disturbances in terms of geographical distribution.
Pilot Project of EGLA Application on 70 kV OHL Parakan-Kadipaten
a. Transmission Line Lightning Arresters
One of the reasons for choosing EGLAs is cost savings. In addition, EGLAs also have a longer lifetime than NGLAs because there is no ageing due to exposure to continuous leakage currents. The spark gap is designed to work below the insulator’s basic insulation level. Installation on the line is carried out by removing the arcing horn to ensure the EGLAs work properly and fulfil EGLAfo < LIWLinsul. The gap on an EGLA must be appropriately designed to work only against lightning overvoltage. When gap discharge requirements are met at switching overvoltage, when there is damage to the SVU, the line will potentially trip during the energizing process. In EHV voltage systems, EGLA installation is generally not recommended to be stand-alone because there is a need to prevent overvoltage switching. Thus, EGLAs are most suitable and effectively installed on systems up to 150 kV. Table 3 shows the specifications of the EGLAs installed on the 70 kV Parakan-Kadipaten OHL.
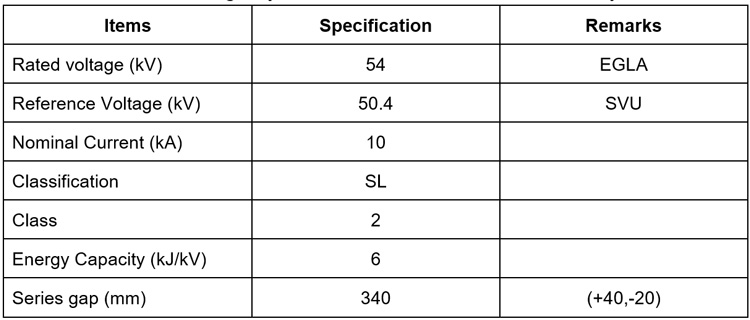
A total of 80 sets of EGLA were planned to be installed alternately on 28 towers of this line. Their configuration is shown in Table 4. Installation considerations were based on supplier experience from installing EGLAs on 20 kV systems and also took account of tower construction in the field.
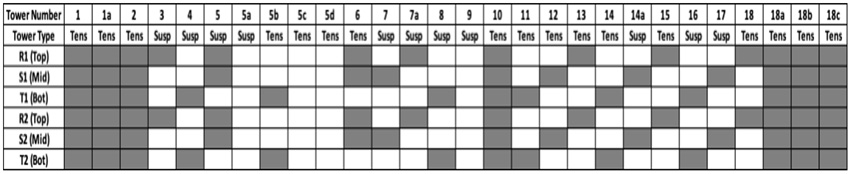
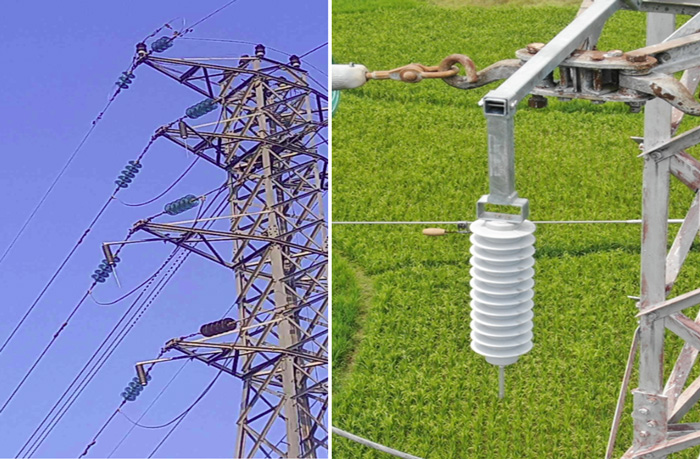
Line surge arresters (LSAs) are prioritized for installation in locations with the following criteria: value of footing resistance is high, shielding angle of earth wire, towers that are often struck by lightning. If there is no budget constraint and to obtain best performance, LSAs should ideally be applied on every phase.
b. Review of EGLA Implementation
Starting Dec. 2022, when EGLAs were first installed until the time of this analysis, there were 3 cases of disturbances caused by lightning. All disturbances occuring on the Parakan Kadipaten Line were triggered by short-circuit of insulators. Most occured on insulators that did not have an EGLA installed installed.
As mentioned in CIGRE TB 855, in cases where the lightning surge travels along the line from the stroke point, lightning fault can be transferred to adjacent towers with no LSAs either via the OHGW at successful shielding or via the conductor at shielding failure. Therefore, LSA installation on adjacent towers beyond the high lightning risk target section is suggested. Note that LSAs may not protect insulator assemblies on the next tower, given the traveling time of lightning surge across the span between towers.
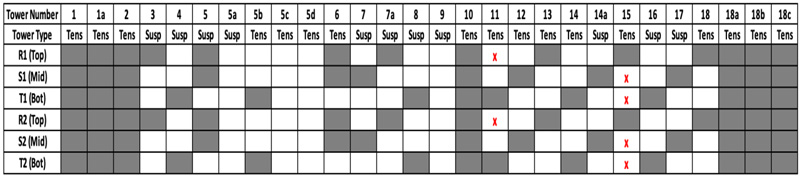
Case 1
A disturbance that occurred on Parakan-Kadipaten#1-2 caused reclosure at the Parakan and Kadipaten Substations and indicated that the relay worked. This was a distance relay for all three phases, R-S-T, zone 1. The chart from Disturbance Fault Recorder at both substations described that there was significant increased current in phase R-S-T-N. There were voltage defects in all three phases which meant that the disturbance was caused by the active system from a lightning surge. Climbing inspection reported that several sets of insulators had flashed over (see Table 6).
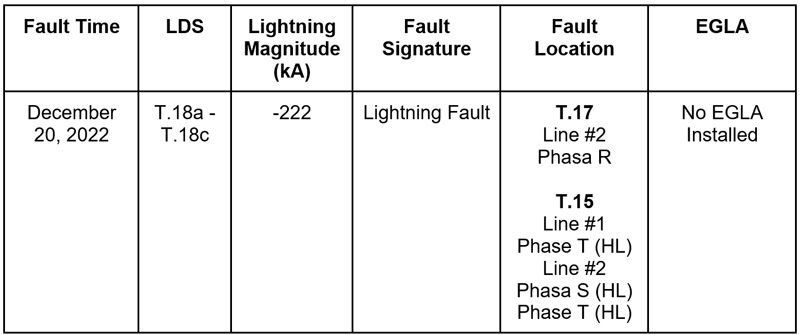
Case 2
A second case involved a disturbance that occurred on the Parakan-Kadipaten #1 Line and which caused reclosure at the Parakan and Kadipaten Substations, again indicating that the relay worked. This was distance relay that worked in phase R-N, zone 1. Based on the Disturbance Fault Recorder at the Kadipaten Substation, there was voltage drop in phase R from 38 kV to 5.6 kV and a current increase from 29 A to 248 A. The Disturbance Fault Recorder at the Parakan Substation recorded that there was voltage drop in phase R from 39 kV into 10.1 kV and an increase in current from 28 A to 592 A. The chart from the DFR showed that the disturbance was caused by a lightning surge.
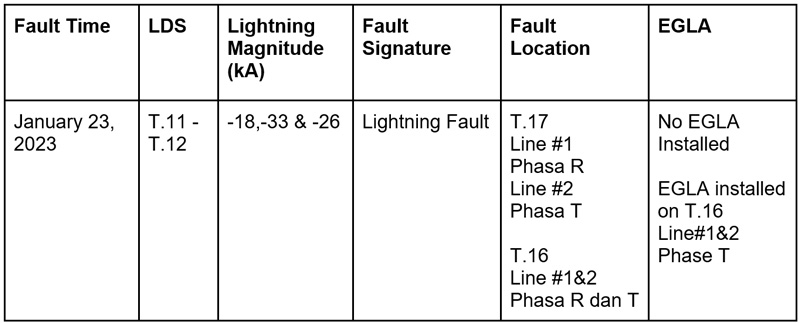
Case 3
A disturbance on the Parakan-Kadipaten #2 Line caused reclosure at the Parakan and Kadipaten Substations, indicating that the relay worked. It was a distance relay that worked in phase R-N, zone 1. Based on the Disturbance Fault Recorder at the Kadipaten Substation, there was a voltage drop in phase R from 38.6 kV to 4 kV and current increased from 30 A to 273 A. The Disturbance Fault Recorder at the Parakan Substation recorded that there was a voltage drop in phase R from 39 kV to 12.7 kV and current increased from 27.6 A to 596 A. The chart from the DFR showed that the disturbance was caused by a lightning surge.
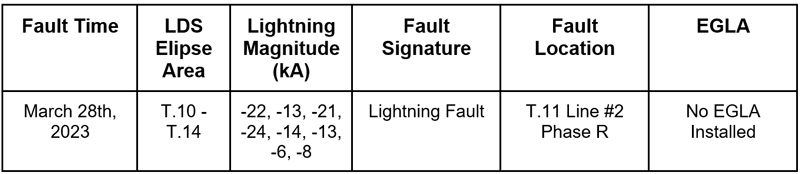
Discussion
The history of disturbances caused by lightning surges on the Parakan-Kadipaten transmission line between 2018-2023 showed that there were 20 such disturbances. Of these, 17 occured before installation of EGLAs while 3 occurred after installation. As seen in Fig. 10, Parakan-Kadipaten Line disturbances can be classified into 2 categories: cluster A (green rectangle) and cluster B (blue rectangle). Cluster A are towers in areas with rice fields while cluster B is in hilly areas. As many as 18 disturbances with 2 times happened in sequence or equal to 90% incident, the disturbance occured in the cluster A area. According to [4], towers in rice field has a higher vulnerability to being struck by lightning. It happens because there are no other structure to be a target which struck by lightning. Disturbances that occurred after the installation of EGLA occurred in the tower 17, 15,16 and 11. According to further investigation, the discharge that occurred was marked by flashover in the insulator. Most of flashover occurred in a place where the EGLA were not installed. Besides that EGLA installation, ageing of the insulator has become one of factors showing that the protection system of the transmission line is not working optimally. This is confirmed by result of dry impulse testing. The test result (see Table 8) indicate that the dry impulse test failed because maximum flashovers allowed is 2.
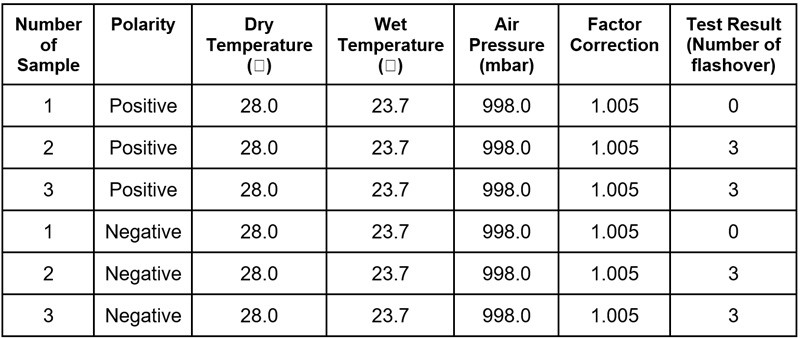
To obtain optimal lightning protection performance on a transmission line, towers that have high critical level need an EGLA installation using the top-bottom configuration.
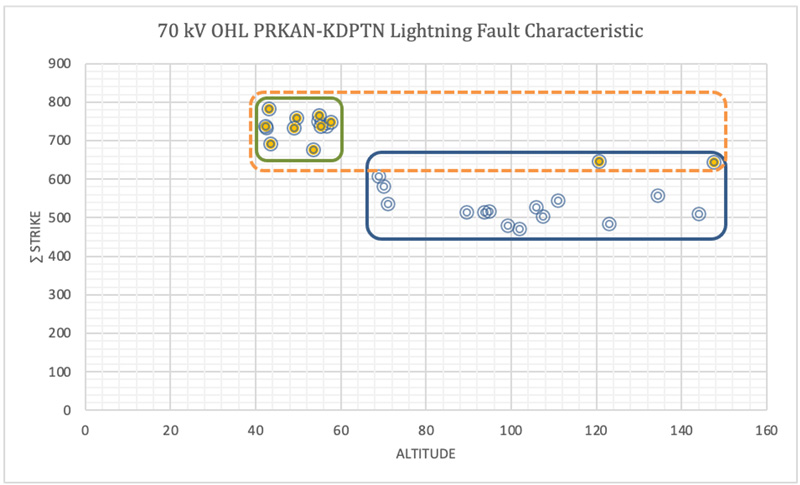
This can be achieved through several options, such as:
1. Adding installation of EGLAs to achieve top-bottom. This can be installed in the cluster A which has high criticality;
2. Relocating EGLAs installed in cluster B and maintaining towers in cluster B with minimum configuration and keeping footing resistance low (<10 Ohm);
3. Replacing ageing insulators to achieve an optimal protection system against lightning surges.
Conclusions
The 70 kV Parakan-Kadipaten Line was selected for installation of EGLAs to test their effectiveness with a particular design. Over the period from Dec. 9, 2022 to Aug. 2023, there were three disturbances that resulted in short circuits on insulators not equipped with an EGLA.
Based on observations in the first half of 2023, after installation of EGLAs there was a 33% decrease compared to the same period of 2022 under conditions of lightning strikes, which also decreased from 625 to 435.
Lightning performance calculations using Sigma SLP software showed that the existing configurations did not significantly reduce disturbances on the 70 kV Parakan-Kadipaten Overhead Line. Retaining existing numbers, installing EGLAs with a new configuration may provide more significant results.
References
[1] CIGRE WG C4, Procedures for Estimating the Lightning Performance of Transmission Lines – New Aspects, no. June. Paris: CIGRE, 2021.
[2] PT.PLN (Persero), “70 kV OHL of Parakan-Kadipaten Lightning Detection System Report,” Cirebon, 2023.
[3] CIGRE 855, Effectiveness of line surge arrester for lightning protection of overhead transmission line, no. December. 2021.
[4] IEEE, Guide for Improving the lightning performance of Transmission Lines. 1997.
[5] R. Bhattarai, R. Rashedin, S. Venkatesan, A. Haddad, H. Griffiths, and N. Harid, “Lightning performance of 275 kV transmission lines,” Proc. Univ. Power Eng. Conf., pp. 2–6, 2008, doi: 10.1109/UPEC.2008.4651622.