Application of silicone rubber composite insulators has increased significantly over the past 35 years but among the key factors behind continued growth is the confidence shown in them by power utilities. This edited 2017 article, contributed to INMR by Prof. LIANG Xidong, and YAN Zhipeng of Tsinghua University in China, explored key issues in this regard. It proposed that IEC test methods and technical standards may not always be sufficient and in some cases need important modifications. It then reviewed test methods used in China – the largest single market for these insulators. Moreover, because of different operating conditions, it explained that specific technical requirements need to be developed for different types of composite insulators, in particular station insulators. Finally, it recommended improved materials and maintenance techniques.
The electric power industry in China experienced rapid increase since the 1980s and, with recent development of UHV AC and UHV DC lines, there has been a surge in electricity generation as well as in the length of the country’s overhead network. Figs. 1 and 2 depict growth in generation and length of overhead transmission lines from 1980 to 2016 and from 1985 to 2016 respectively.
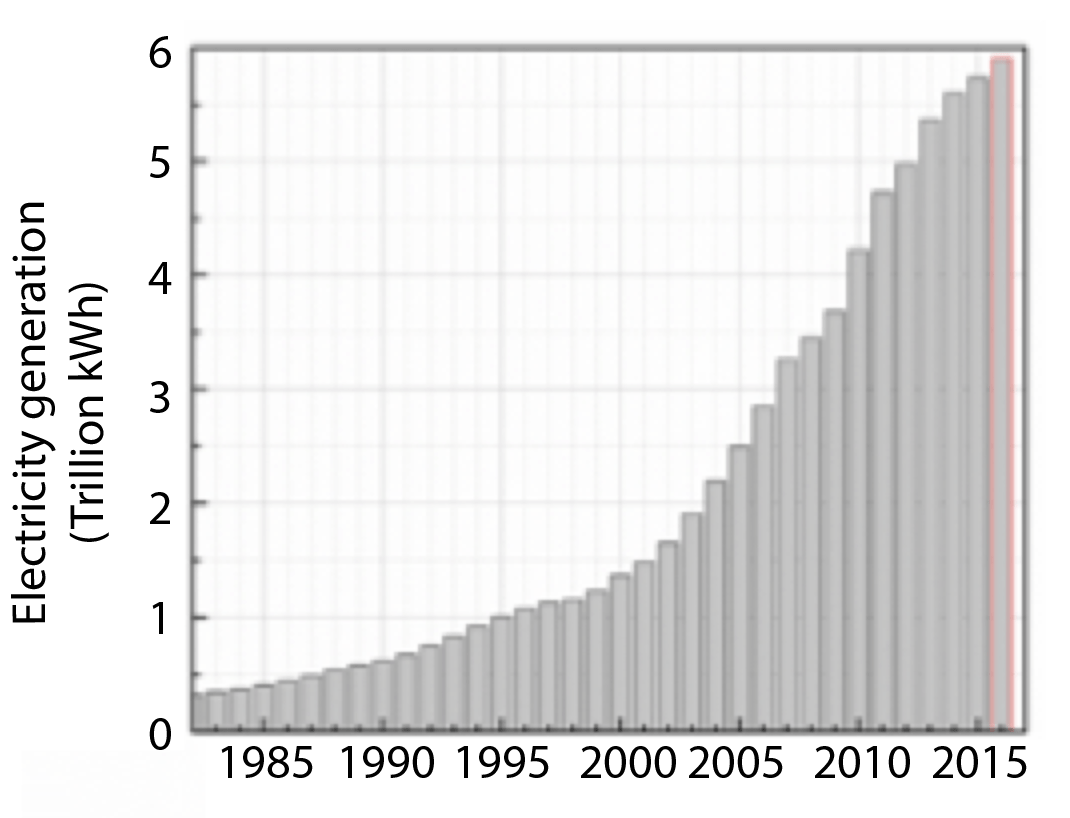
Development of insulators cannot be viewed separately from development of the power sector as a whole. For example, rapid growth in length of overhead lines and the move to higher voltage levels brought not only a huge increase in demand for insulators but also new and greater requirements in terms of their performance. Because of advantages in regard to superior wet and pollution flashover performance, high strength to weight ratio, easier transport and installation, resistance to vandalism, etc., application of silicone rubber composite insulators in China realized significant growth.
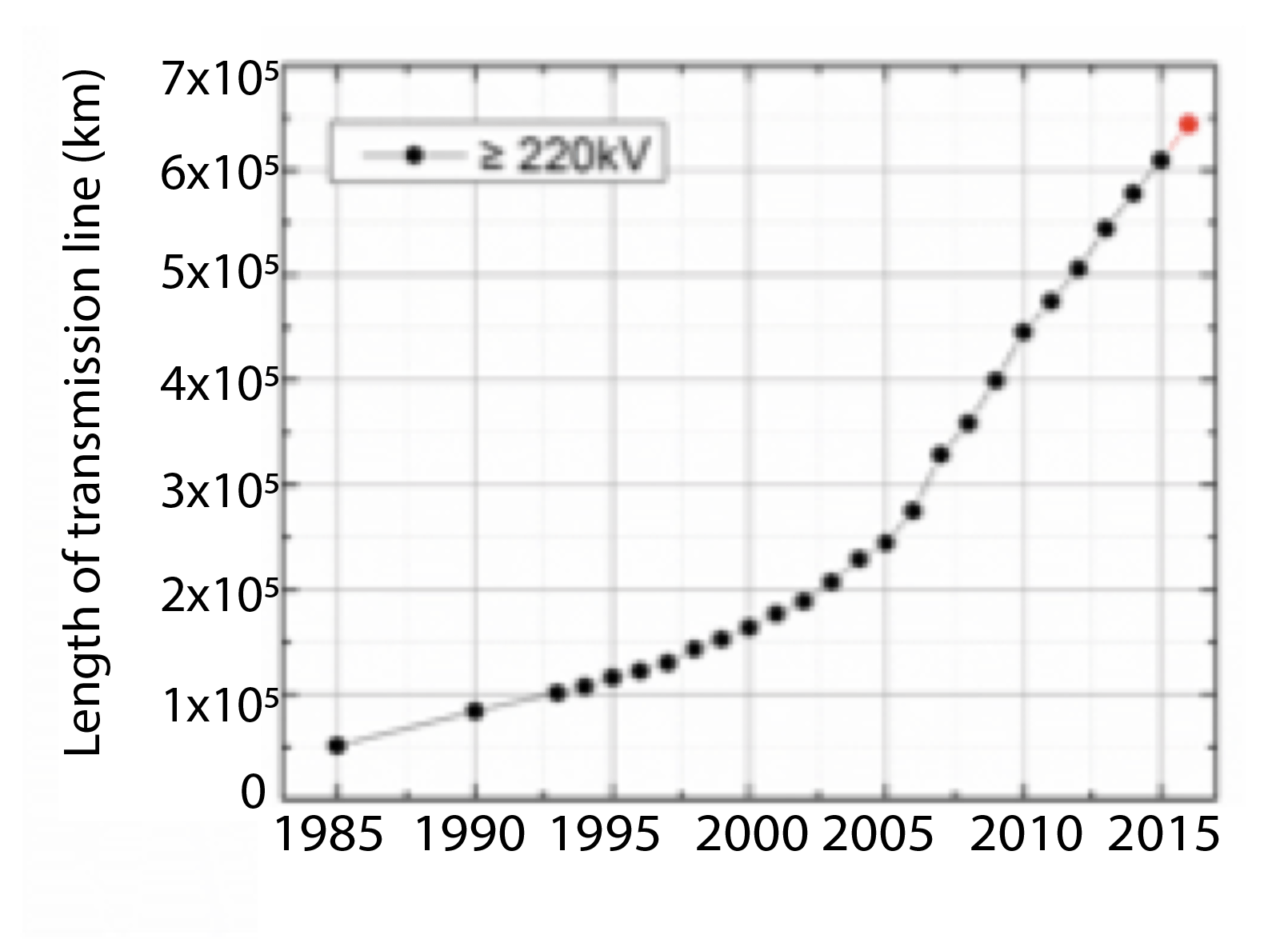
Statistics on number of line insulators purchased by the State Grid Corporation of China (SGCC) from Jan 2012 to Sept 2017 are shown in Fig. 3. This data covered the range 110 kV to 1000 kV AC, and ±800 kV DC as well as 70 kN to 550 kN SML (in the case of porcelain, glass and silicone composite insulators) and 1000 kN SML for composite insulators. During this period, the SGCC purchased 34.1 million porcelain insulators, 25.3 million glass insulators and about 5.13 million strings of silicone composite insulators. If converted to number of strings to allow for direct comparison, porcelain accounted for approximately 2.48 million strings and glass for about 1.85 million strings. The proportions of porcelain, glass and composite insulator strings purchased were therefore 26%, 20% and 54%, respectively. Moreover, among newly installed insulators, the number of composite insulator strings was more than the sum of the other two types of strings. This confirmed that China has become the first country where silicone rubber insulators dominate EHV as well as UHV overhead lines.
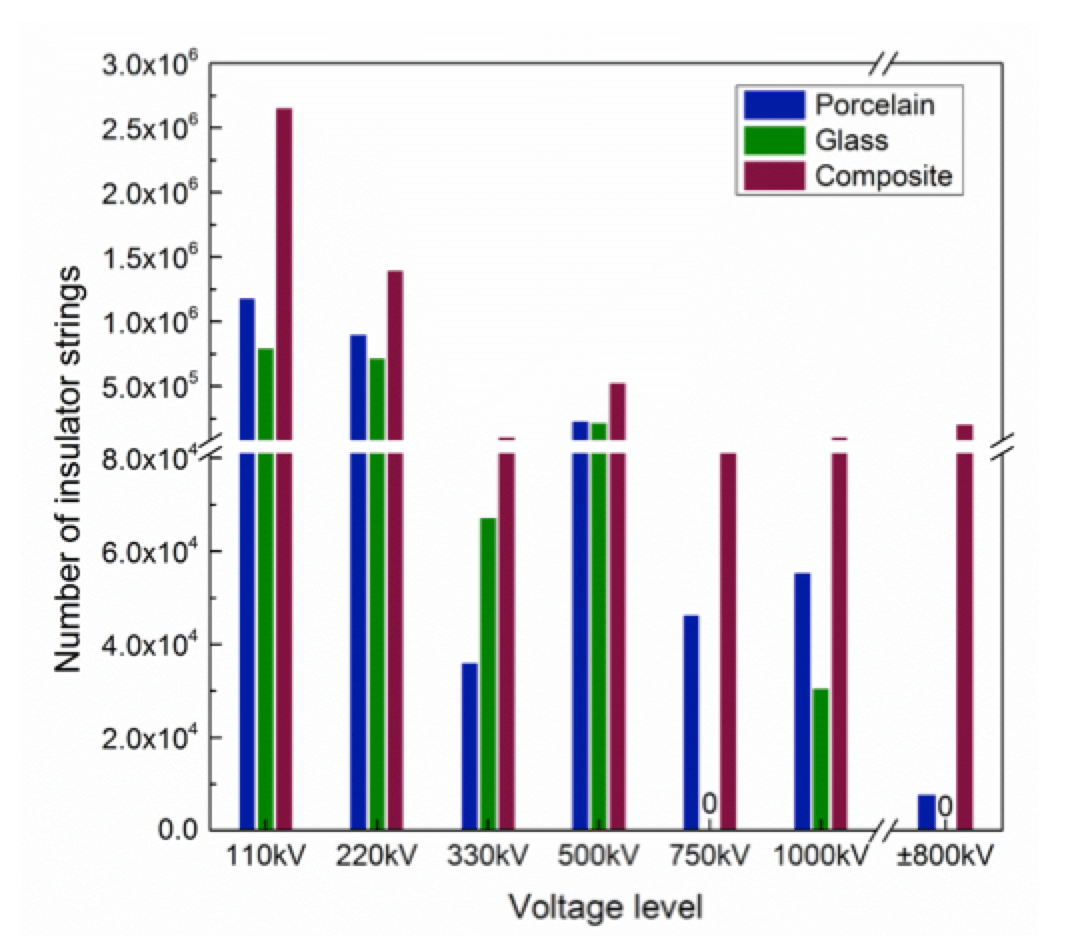
Composite insulators also realized significant increase in use worldwide and, based on trial applications, now dominate all UHV lines. Moreover, major improvement has been achieved both in their manufacture and testing. Still, given the rapid development of this technology, the key issue for the future of composite insulators is that power utilities remain confident in their expected service life and performance. That means future development of composite insulators (as shown in Fig. 4) will depend on: availability of suitable test methods to verify long-term performance; establishing different requirements when it comes to station insulators; and developing the best materials and maintenance techniques.
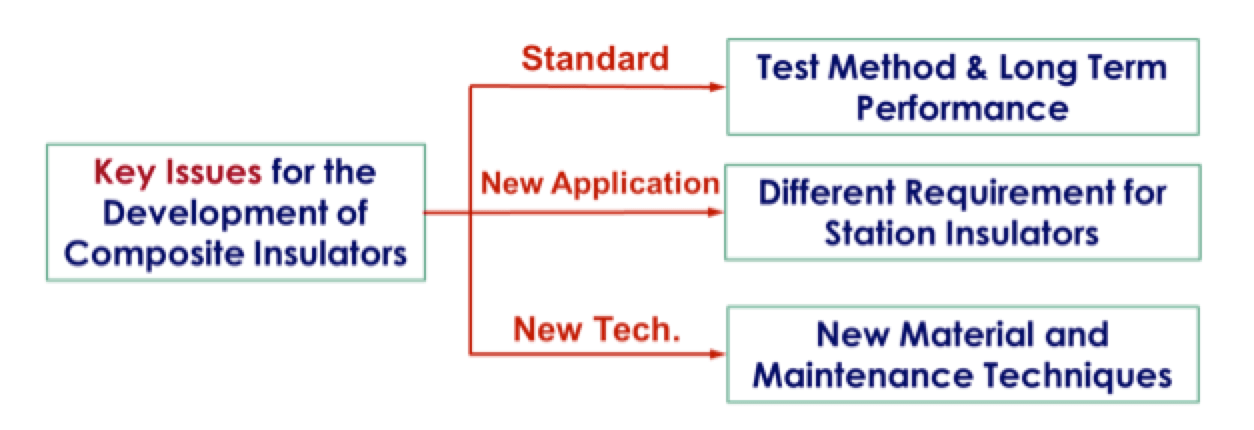
Test Methods & Long Term Performance
Among the technical standards published to ensure performance of composite insulators is IEC 62217, Polymeric HV insulators for indoor and outdoor use – General definitions, test methods and acceptance criteria, whose scope covers polymeric insulators where the insulation body consists of one or more organic materials. Polymeric insulators covered by this standard include both solid and hollow core insulators intended for application on overhead lines or for indoor and outdoor equipment with rated voltage greater than 1000V. Unfortunately, test methods do not necessarily guarantee satisfactory performance over the full service life of an insulator. For example, some composite insulators that pass standard tests offer much different performance when in operation, even in similar environments. One specific problem is that such tests are not yet sufficient to adequately screen for interface quality, rod quality and quality of the shed-to-housing interface.
Tests on Rod Material
The recent IEC standard only specified a dye penetration test and a water diffusion test for the rod material. But rod damage in service continues to account for a large proportion of insulator failures, such as brittle fracture and decay-like fracture. For example, as shown in Table 1, rod failure has been a leading cause of service failures, accounting for some 22.3% of all such incidents. This suggests that rod quality is far from satisfactory and that more test methods are needed to guarantee quality and prevent such damage.
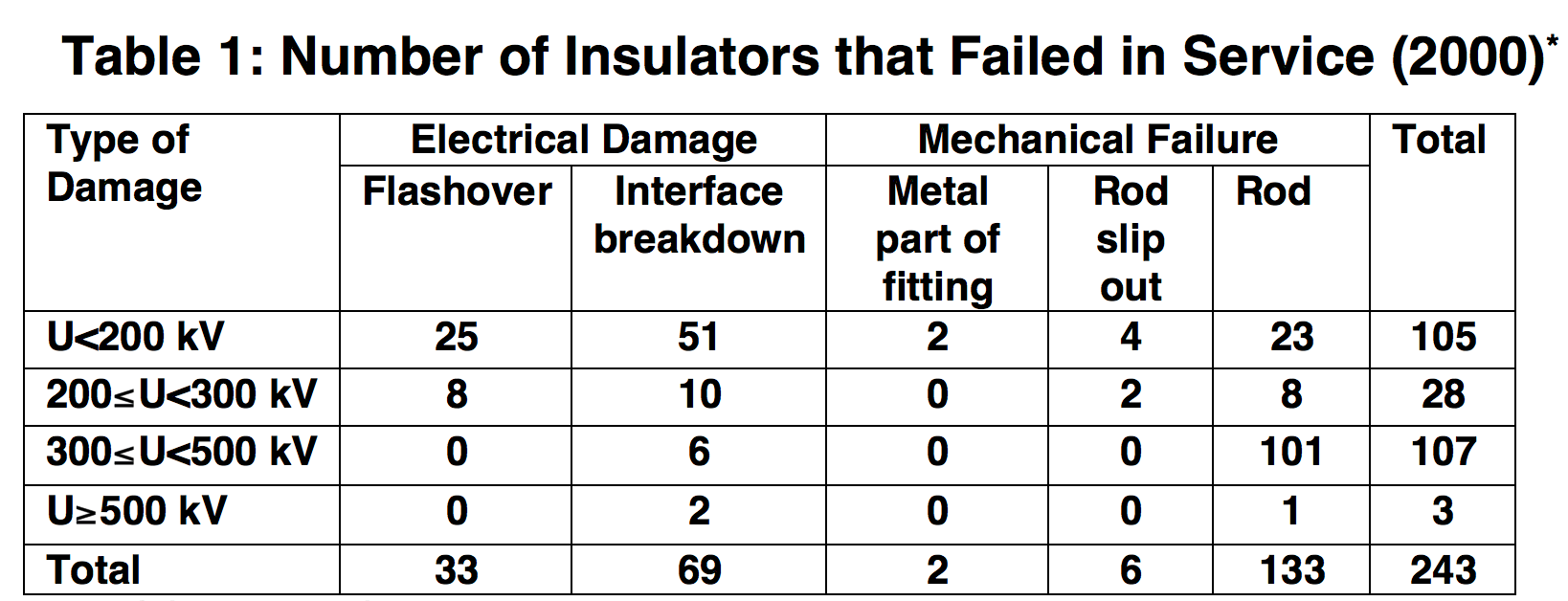
While brittle fracture failure occurs among only a very low proportion of all composite insulators in service, it could result in sudden conductor drop with serious consequences. Usually, insulators so affected fail at low mechanical loads and after only several months or years of service. The fracture surface in such cases is typically flat and smooth, as shown in Fig. 5.
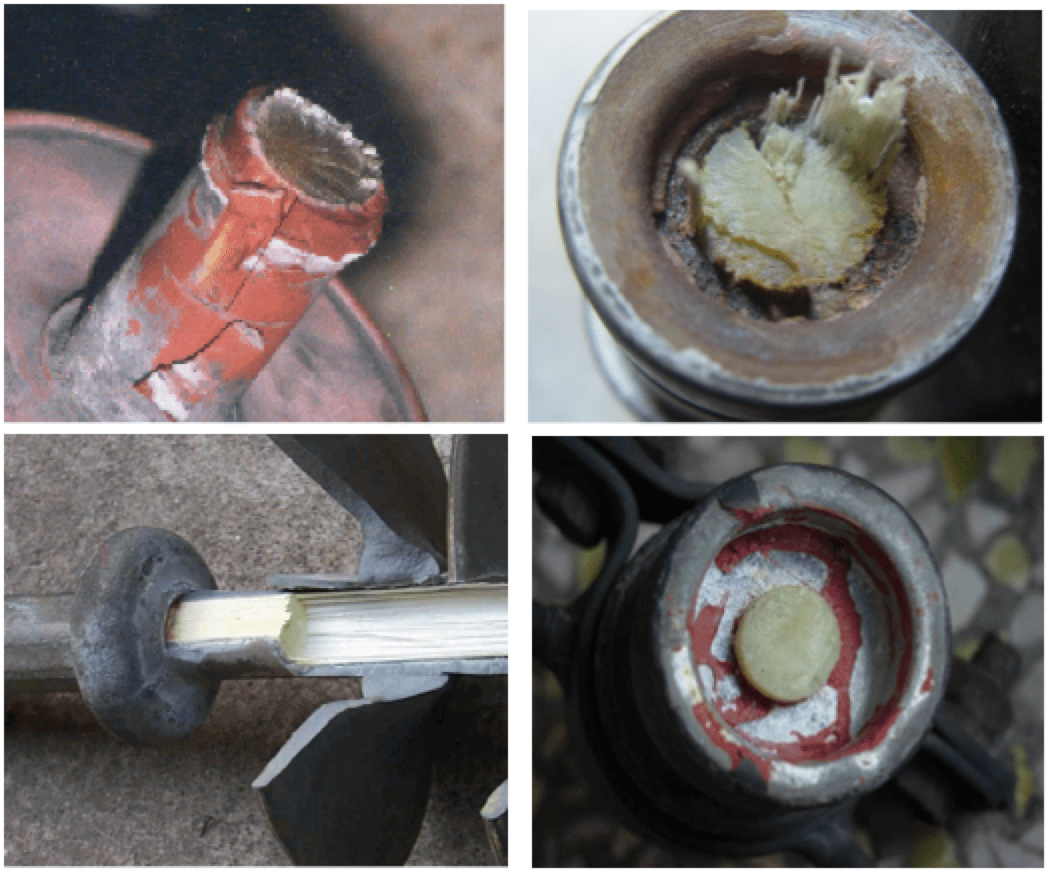
While brittle fracture has been reported in many countries, from Europe to China, there is still no relevant IEC test. Since the mechanism behind such failure is clearly stress corrosion, such a test has already been developed and adopted in the Chinese electric power industrial standard DL/T 810-2002 (revised in 2012). Specifically, this standard requires that the FRP rod must be acid proof (i.e. use boron-free glass). Since publication of this standard, there have been no reports of brittle fracture among newly installed composite insulators with acid proof rods. This serves to confirm that specifying such rods eliminates this risk. Decay like fracture is another type of rod failure mechanism. Although incidence of this phenomenon is even less than brittle fracture, the problem is as serious. Affected insulators also fail at low mechanical load after only a few years of service. But in this case the fracture surface is crisp, like dead wood, as in Fig. 6. Moreover, chalking has been seen on the surface of affected insulator sheds and it was found that glass fiber separated from the resin in the rod. In China, this problem has occurred mainly on 500 kV lines and it is worth noting that such failures have increased over the past 10 years.
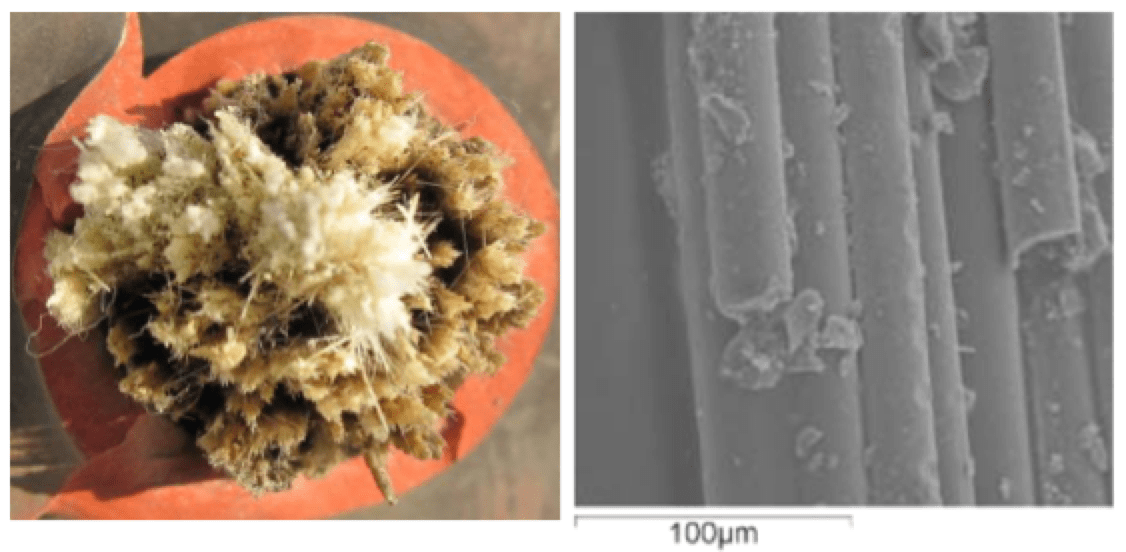
The test in past IEC standards cannot guarantee prevention of decay-like fracture since some rod materials have passed yet subsequently failed in operation. Moreover, the exact mechanism behind these is still not fully clear. Experience suggests that such fractures can be detected before failure using IR imaging to look for a local temperature rise. But this allows only detection. In order to prevent the problem, more screening tests need to be conducted on the rod material.
Tests on Shed Material
In the IEC standard, tests on the housing/shed consist of 4 parts: hardness test, accelerated weathering test, tracking and erosion test (1000 h salt fog test); and flammability test. According to experience at the SGCC as well as at the China Southern Power Grid, these tests need improvement. For example, recent IEC standards did not consider hydrophobicity and its transfer. Also, the test method for tracking and erosion needed to be improved while the multi-stress test did not seem suitable for materials such as silicone rubber.
Hydrophobicity and its transfer are the most important properties for HTM (hydrophobicity transfer material) composite insulators but still not mentioned in IEC. In this regard, Tsinghua University has proposed a test method for hydrophobicity and its transfer in the case of silicone rubber insulators and this has been used in China for 15 years now. Put forward in local standard DL/810-2002, the test is divided into 4 parts: a hydrophobicity test, a loss of hydrophobicity test, a recovery of hydrophobicity test and a transfer of hydrophobicity test. Each has its own test method and acceptance criteria.
As for the recent IEC tracking and erosion test, there are still questions about method and effects. For example, salt spray directed toward the insulator in the 5000h salt fog test seems misleading in terms of the technical requirements actually placed on a composite insulator applied in severe service conditions. A rotating wheel dip test is another option for assessing tracking and erosion performance but unfortunately shows relatively large scatter in results at different laboratories. There is also the issue of testing much larger insulator diameters as is now necessary by ongoing development of composite station insulators. Also, with increasing application of composite insulators on HVDC lines and at converter stations, a tracking and erosion resistance test under DC voltage has been specified in China but is still not clear in IEC.
To guarantee long-term performance of shed material, an accelerated weathering test has been recommended within IEC. Unfortunately, this has no effect on silicone rubber based on experience to date in China. Specifically, the current test does not sufficiently simulate the effect of non-soluble pollutants and the hydrophobicity change process for silicone rubber material. A modified 5000h test procedure – the THU 5000h test procedure – has therefore been proposed. This test procedure (see Fig. 7) contains two major modifications: 1. replacing the salt fog with mixed contamination fog (NaCl and kieselguhr) and 2. providing sufficient time for hydrophobicity loss and discharge activities in the fog chamber and also sufficient time for hydrophobicity transfer in the UV chamber (as per Fig. 8).
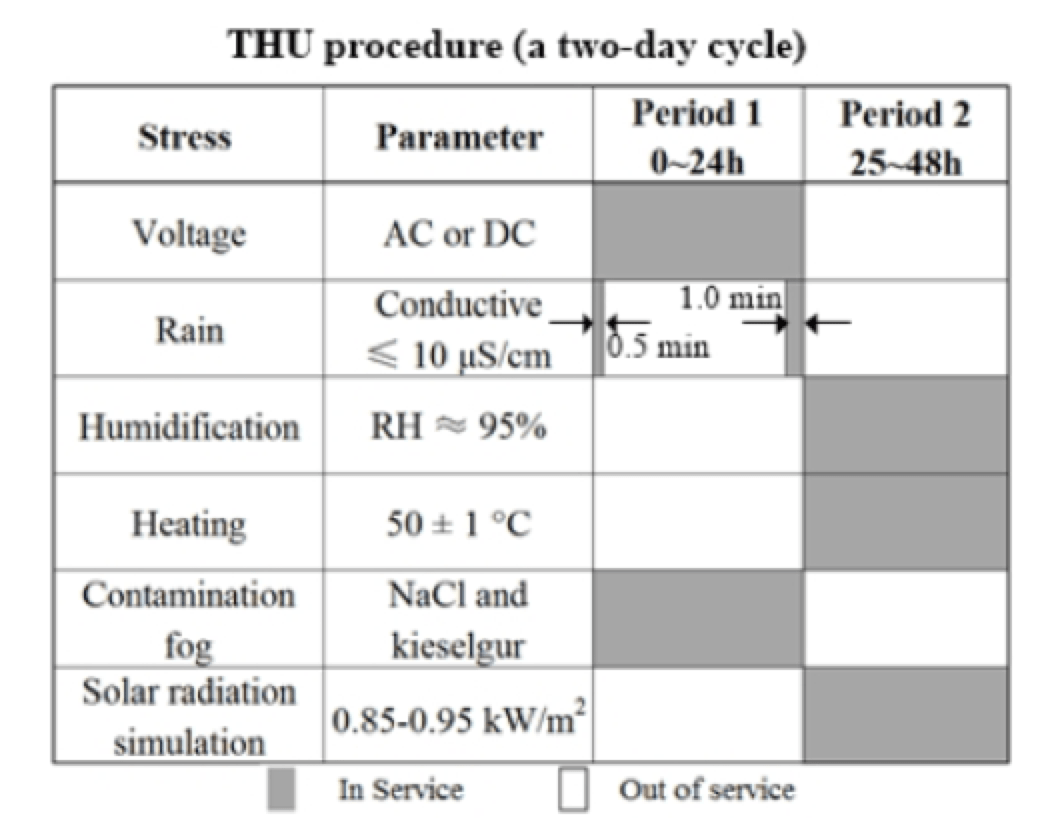
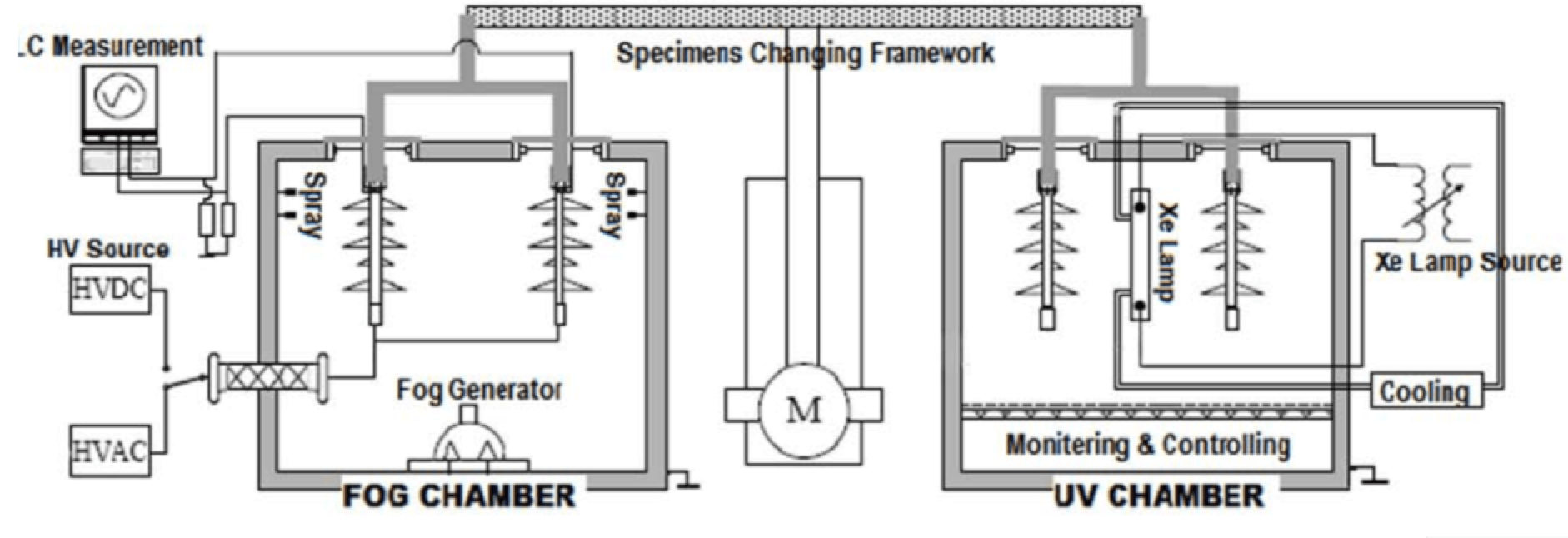
Tests on Interfaces
Poor interface adhesion or failure of interface coupling in a composite insulator can result in different types of problems, e.g. interface puncture, local temperature rise caused by interface leakage current and eventually decay-like fracture. Moreover, there may also be a relationship between weak interface adhesion with brittle fracture. There is evidence that this may be a growing problem since the interface between rod and shed is always the critical area of a composite insulator. IEC 62217 and other standards provide tests for interfaces and connections including the reference dry power frequency test, the pre-stressing and verification test. A steep-front impulse voltage test (1000kV/ms) is the main test of performance. A limited test cannot guarantee performance of the interface over tens of years of operation outdoors. In fact, failures of the shed–rod interface have been documented going back to a survey in 1990 where 203 insulators were found to have failed because of problems in the shed-rod interface (see Table 2). Similar findings were reported in another survey conducted in 2000 (see Table 1), where 69 insulators were reported to have failed because of interface breakdown. The interface problem is significant if looked at over the past 30 years. Indeed, a survey in China over this time frame showed similar results.
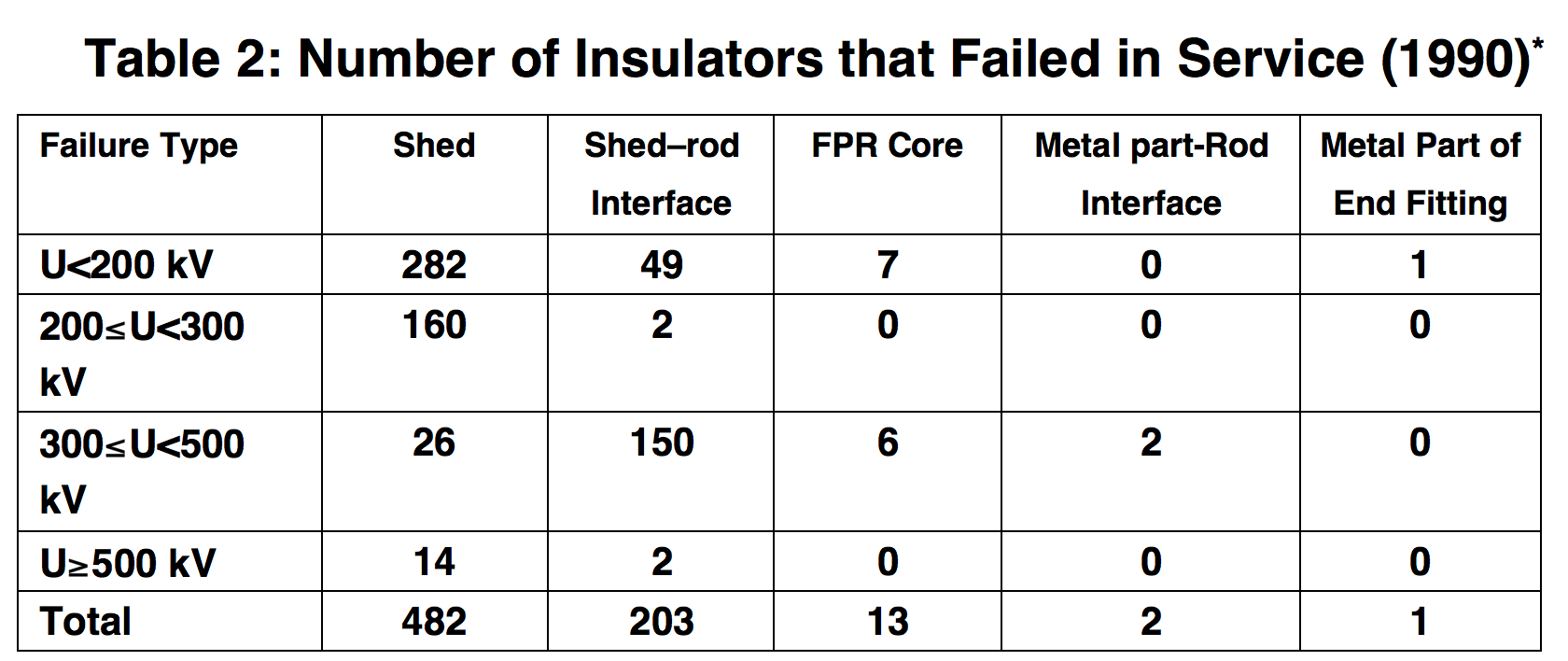
The steep front impulse voltage test according to IEC 62217 and IEC 61109 was not able to guarantee interface quality and this test was therefore modified. Applied voltage was changed from 1000 kV/ms to 30 kV/cm and this meant a higher requirement for interface performance. Among the possibilities for a modified test method for the interface is resistivity measurement used to test the dielectric property between silicone rubber and FRP rod.
Price & Long-Term Performance
Due to intense competition, the relative market price of silicone rubber insulators versus other types is highly attractive these days. This is especially so in the case of higher voltages. For example, cost comparison between porcelain, glass and composite insulators of from 110 kV to 1000 kV AC as well as ±500 kV and ±800 kV DC in China is shown in Figs. 9a & b, respectively. Insulator cost per string is calculated based on application in a general service environment. It can readily be seen that silicone rubber composite insulators are always far less costly than either porcelain or glass insulators and that this cost advantage increases with higher voltage or mechanical level. The cost differential is even greater in the case of DC.
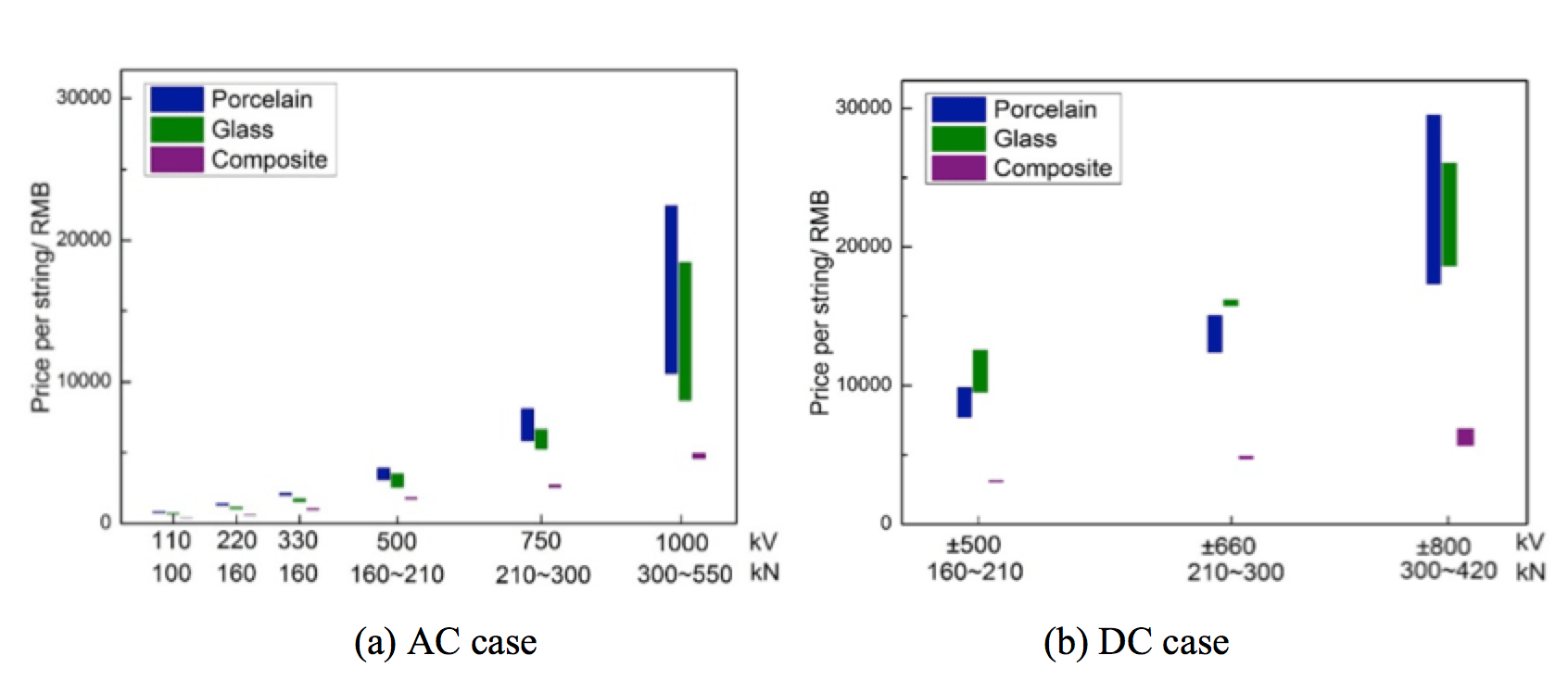
While a lower acquisition cost is one of the advantages offered by silicone rubber composite insulators, too low a price may suggest possible increased risk of lower quality and inferior long-term performance. Based on Chinese service experience with HTV silicone rubber, some insulators have operated without problem for more than 15 years but many early generation products had to be replaced within a decade. Since number of years in service is now often less than 20 years, this could impact user perceptions and confidence in composite insulators. The new generation of SR composite insulators is expected to operate for longer. But since utilities want to purchase reliable as well as less costly insulators and since manufacturers need to control cost of production, current insulator standards are not sufficient to meet all these interests.
Recommended Modifications to Standards
Modification to test methods in the current standards is urgent but it is also important to first define the technical requirements that must be met. A technical standard has to guide the insulator manufacturer to correctly understand the requirements of outdoor insulation and to avoid any random changes in composition or production process. At the same time, another requirement is to guide the utility procurement process. Quality of composite insulators is determined by material, design and by the manufacturing process. Accordingly, in order to improve performance, tests on material, design and manufacturing may need to be incorporated into future standards. In China, for example, DL/T 810-2002 has been used for 15 years now and marked changes from the first to second generation silicone composite insulators. As mentioned, a stress corrosion test was conducted to prevent brittle facture, while both a hydrophobicity and a hydrophobicity transfer test were defined for HTM materials. Other elements included a tracking and erosion test for HVDC as well as several material tests on the silicone rubber and the FRP rod. Moreover, a steep-front impulse voltage test (30kV/cm) was modified to meet the requirements of UHV AC and UHV DC in China.
Different Requirements for Station Insulators
Substations are a major new application area for composite insulators although for the past 20 years their volume and proportion of application was far less than for line insulators. That situation is now changing. While one of the reasons silicone composite insulators are now being used at substations is because of their success on overhead lines, line insulators and station insulators operate under different conditions. The location of line insulators is dispersed along the full length of a line, making monitoring them a challenge. Moreover, because the unit value of a line insulator is relatively low, monitoring all of them for ageing is not regarded as necessary. Similarly, repairing any localized damage found is usually not cost-effective since the connection between insulator and tower is relatively simple and line insulators can be replaced individually or in batches. This makes it possible to rely on sampling some insulators within the population of a line or even some portions of insulators for monitoring and research purposes during service. By contrast, the comparative concentration of station insulators offers an advantage in carrying out all kinds of monitoring. Moreover, because the individual value of a station insulator is high, it is worthwhile developing monitoring techniques and any localized damage found is worth repairing. At the same time, sampling for research during service is almost impossible. A substation insulator is often applied to high voltage equipment so it is typically not convenient to replace. For example, because of different features, station insulators can be divided in two types: Type I – easy to replace; and Type II – difficult to replace. The main example of a Type I insulator is the busbar station post. Type II insulators include GIS bushings, wall bushings, power transformer bushings, hollow insulators for CTs and PTs, and hollow housings for ZnO surge arresters.
Lifetime Requirement for Station Insulators
For Type II station insulators that are difficult to replace during service, close attention must be paid to service life and long-term performance. For example, serious ageing has already been observed on a wall bushing that has operated for only 15 years (see Fig. 10). A large area with cracks and chalking phenomenon appears on shed surfaces of the portion outdoors. These surface cracks were found mainly in the upper part of the housing (i.e. exposed to sun). Chalking of the silicone rubber appears on the high voltage side. Because of this chalking, the mechanical strength of the surface has decreased significantly.
Moreover, in most areas, such as the HV and middle portions, the hydrophobicity classification decreased to HC7 and water on the surface reveals a hydrophilic state. The price of the shed material is much less than that of the wall bushing itself and replacement cost is also much higher than the shed material cost. Because sampling is not possible during service, the ageing process and its various contributing mechanisms are more difficult to determine. This example highlights that, compared with overhead lines, monitoring the ageing performance of composite insulators at substations is even more important.
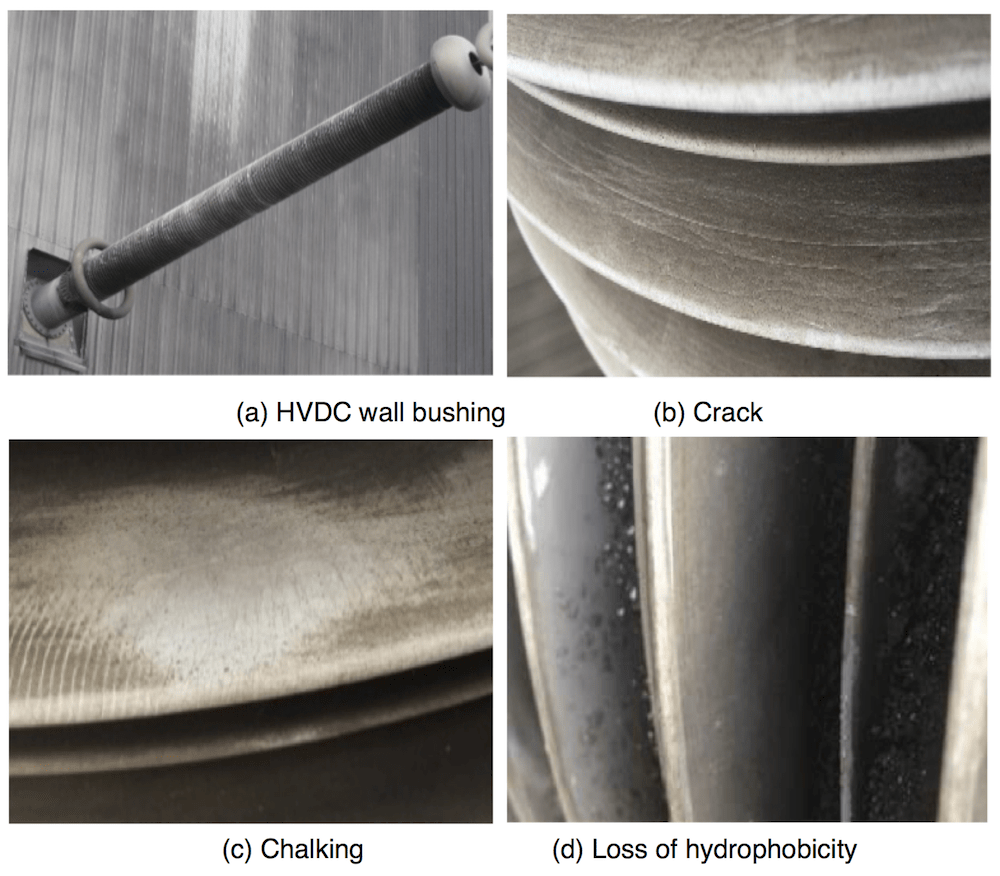
Test Methods for Station Insulators
A substation insulator operates under different conditions from one used on an overhead line. Moreover, different station equipment operates under own particular conditions in regard to electric field distribution, bending and torsional rigidity and temperature. It is therefore better to apply different tests to inspect these different insulators and also to set different acceptance criteria for each.
New Material & Maintenance Techniques
Materials with Super-Hydrophobicity
New super-hydrophobic materials are especially attractive for outdoor insulation and researchers have come up with various methods to create these. For example, Tsinghua University used a laser-ablated template and fluoroalkyl-silane-modified composite coatings to prepare a specific microstructure and nanostructure on a silicone rubber surface (see Fig. 11).
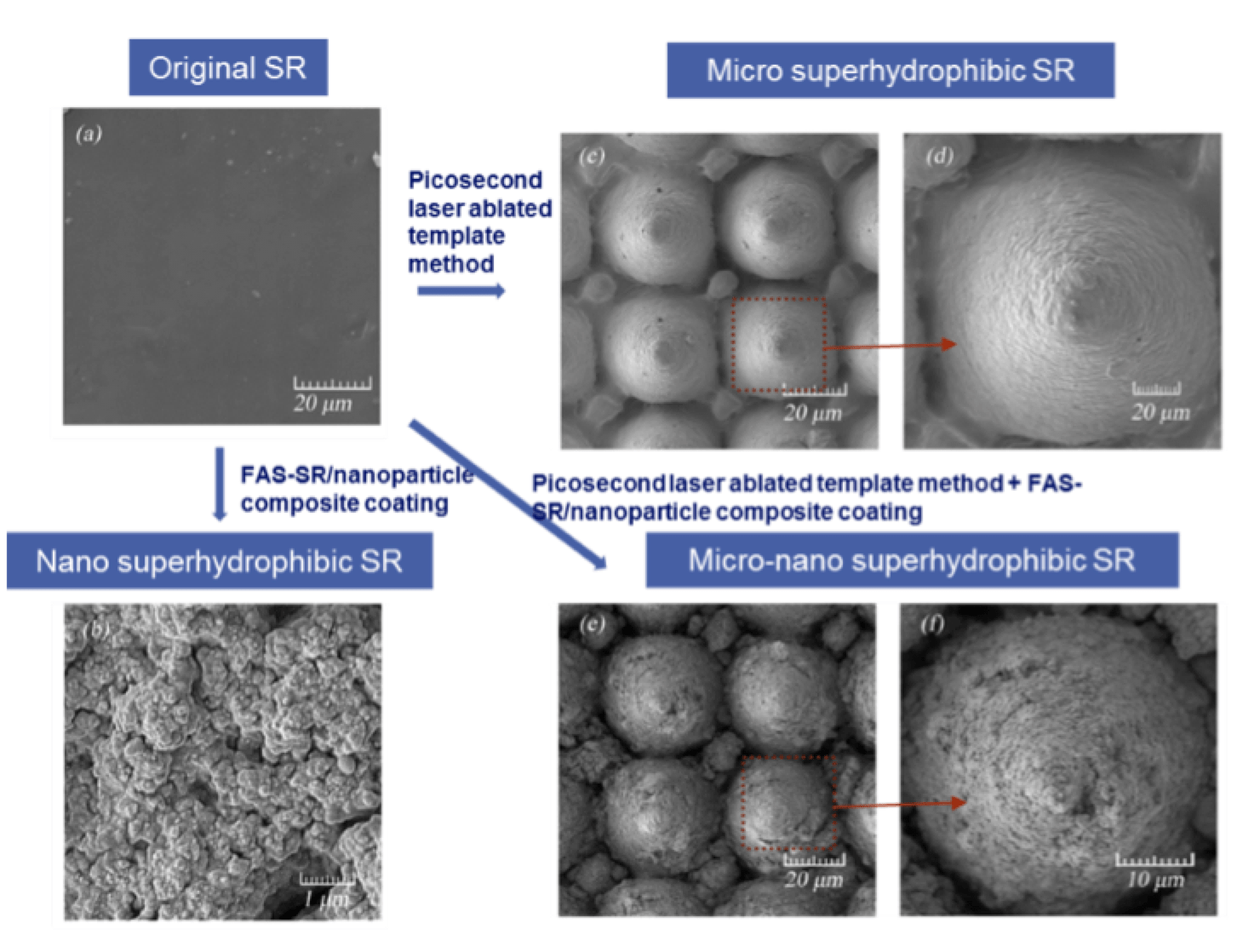
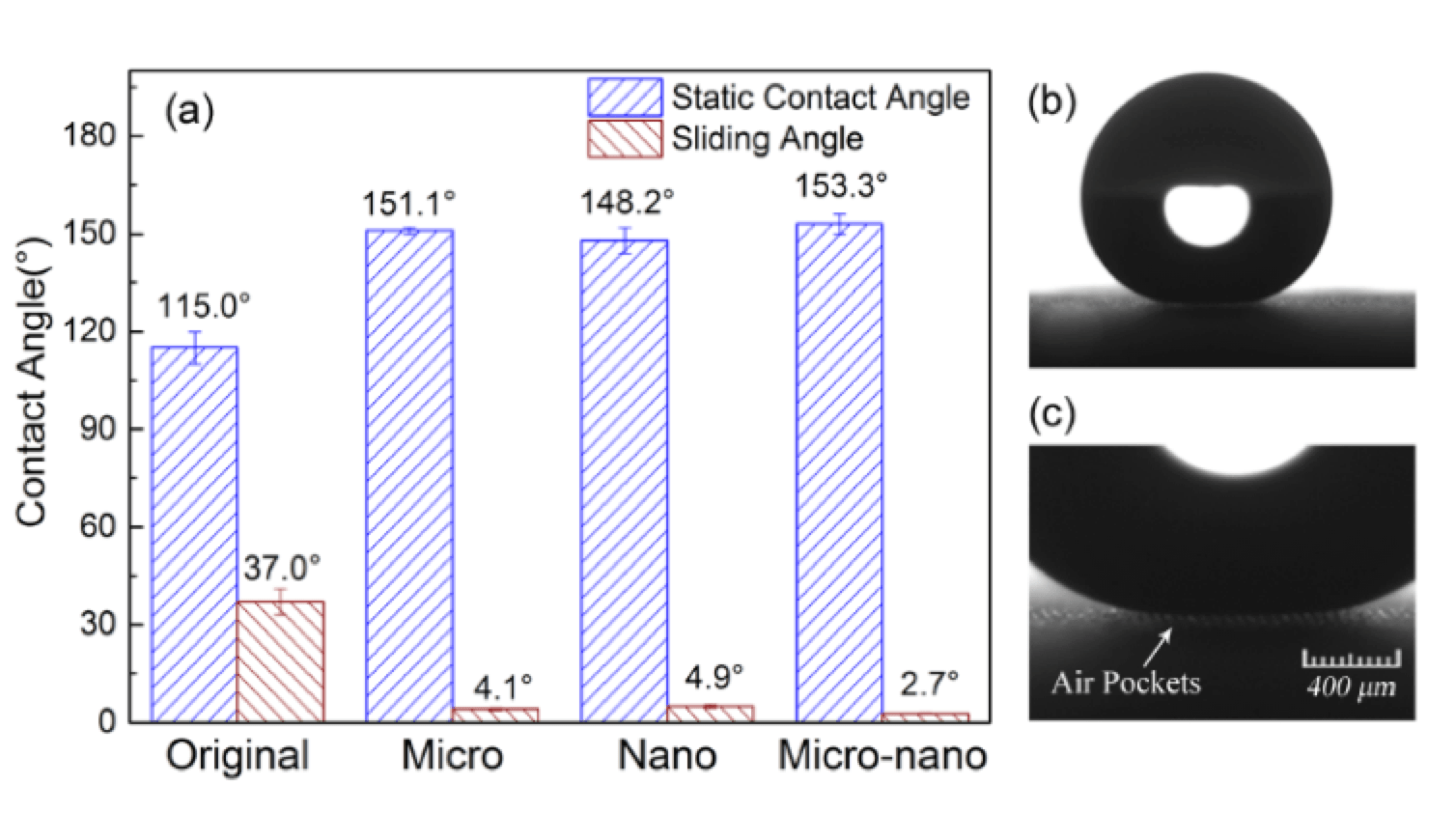
Fig. 12 shows the results of static contact angle and sliding angle measurements on HTV silicone rubber surfaces with different micro, nano, and micro-nano hierarchically textured surfaces. The static contact angle of water drops on the unaltered HTV silicone rubber sample was 115 ± 0.7° (i.e. classified as hydrophobic). In the case of micro structured HTV silicone rubber surfaces, this increased to 151.1 ± 1.7°, while with sliding angle to 4.1°. Nano-structured HTV silicone rubber surfaces offered a static contact angle of 148.2° and a sliding angle of 4.9°. The micro-nano structured HTV silicone rubber samples yielded the highest static contact angle,153.3°, and a very low sliding angle of 2.7°. A super-hydrophobic silicone rubber shows an excellent water repellency property when water droplets impact it under electric field. The surface can also be used to solve the problem of uneven field distribution due to surface water film and electric field enhancement due to water droplets. Large area such samples have successfully been prepared and it has been found that this type of surface also offers anti-icing as well as self-cleaning properties. Clearly, high volume production of long-lasting, super-hydrophobic surfaces will be an important future development in the field of outdoor insulation.
Other New Materials
There are also other developments to watch, such as nano-fillers and modification of fillers. These may provide better solutions to improve the shed/sheath of composite insulators or the FRP rod by improving or replacing the fiber or matrix materials. Another promising development is improvement of the critical interface between sheath and rod using new coupling agents or new treatment methods. Other possibilities include self-diagnosing and even self-healing materials.
Maintenance Based on Non-Contact Monitoring
On-line monitoring represents a huge challenge for a power grid. Development of industrial robots will be a promising way to conduct non-contact monitoring of insulators, such as looking for any localized heating that could be caused by surface leakage current, internal defects or degradation of the housing-rod interface. Currently, a localized temperature rise is considered related mainly to degradation of an interface and a sign of the early stage of decay-like fracture. Non-contact monitoring methods such as IR inspection from helicopters or unmanned aerial vehicles allow such localized heating to be detected so that affected insulators can be replaced in a timely manner, based on level of temperature rise.
Maintenance Based on Big Data
Information technology will certainly change development of power grids. In the case of composite insulators, two possible properties might meet some of the needs of the future smart grid. The first is large-scale, real-time on-line condition monitoring, both at substations and on overhead lines. A new function could be added to composite insulators, namely obtaining and transmitting data. Another is failure prediction based on big data. Every insulator will need to have its own QR code where all information such as manufacturing and material details, time in operation, maintenance information, etc. will be obtained. With such data, quality maintenance will become digital and failure prediction could be based on big data.
Conclusions
Use of composite insulator has increased rapidly over the past 30 years. Looking to the future, there are still key issues for continued development of this technology. Currently, test methods and technical standards are not satisfactory and it will be important to make modifications, not only to improve composite insulator quality but also to increase utility confidence when choosing such insulators. Specific test methods and requirements will need to be developed for different types of composite substation insulators. Also, new materials and better maintenance techniques will both contribute to further development of composite insulators.